Alkaline phosphatase and cardiovascular disease
Historical perspective
Alkaline phosphatases [AP; orthophosphoric monoester phosphohydrolase (alkaline optimum); Enzyme Commission number (EC) 3.1.3.1.] are plasma membrane-anchored enzymes that are widely distributed in nature from bacteria to humans. The enzymes catalyze the hydrolysis of phosphate monoesters from a wide range of substrates as well as a transphosphorylation reaction in the presence of abundant concentrations of phosphate acceptors (1). AP was discovered by Suzuki et al. (2) who named the enzyme “phytase” (because the enzyme released phosphate by hydrolyzing phytic acid). A series of studies by Robison and colleagues in 1920s made a great contribution to biochemical characterization of AP including postulation of its physiological function. In 1923 Robison (3) found that bone extracts from rabbits and rats but not extracts from non-ossifying cartilage hydrolyzed hexosephosphoric ester to liberate phosphate. Subsequently, the enzyme which was present in bone was named “monophosphoric esterase” by Robison and Soames (4). In 1924 Kay and Robison (5) measured the enzyme activity in human blood and called it “bone enzyme”. Subsequently, terms “phosphatase” or “bone phosphatase” were used by Martland and Robison (6). Notably, Robison (3) hypothesized that AP may participate in bone physiology by increasing phosphate availability needed for bone mineralization—a hypothesis that has stood the test of time. Historical aspects of AP discovery, characterization and evolution of laboratory assays used for its measurement have recently been reviewed by Siller and Whyte (7). AP has been considered the most frequently assayed enzyme in medicine (7).
Structure and function
Human AP consists of four different types: the tissue non-specific AP (TNAP) and three tissue-specific AP (intestinal, placental and germ-cell types). TNAP is expressed in many tissues throughout the body but its highest activity is found in bone, liver and kidney (also known as bone/liver/kidney AP type). The tissue specific APs are 90% to 98% homologous whereas TNAP is 50% homologous to the other three tissue specific APs (8). TNAP is a homodimeric glycoprotein with each monomer consisting of 524 amino acid residues (57.2 kDa) (9,10). TNAP has several domains including active site, several mammalian specific domains like long-terminal α-helix domain that is responsible for catalysis, a non-catalytic Ca2+-binding domain and a crown domain that is responsible for uncompetitive inhibition, allosteric properties, heat stability and interaction with extracellular matrix components including collagen (11). The active site has two binding sites for two Zn2+ ions, a binding site for a Mg2+ ion and serine 102 to which phosphate binds (12). TNAP undergoes multiple post-translational changes including extensive N-glycosylation in at least 5 sites (asparagine residues 140, 230, 271, 303 and 430) and at least 1 undetermined O-glycosylation (13,14). The glucan content is highly variable but it contributes at least 20% of the dry weight of most preparations (15). All mammalian AP have five cysteine residues per unit that form two disulfide bonds that contribute to enzyme conformational stability (1). The enzyme is anchored to the plasma membrane via its carboxyl terminal end by a phosphatidylinositol-glycan moiety (16). All four AP are coded by four different genes. The TNAP gene is located at the tip of chromosome 1 (1p36.1-p34) whereas the genes for the other three tissue specific AP are located at the end of the long arm of chromosome 2 (2q34-q37). The complete sequence of TNAP gene is known. TNAP gene is comprised of at least 50 kb of DNA and consists of 12 exons, of which the first and part of the second are noncoding (17,18). There are also two leader exons (exon 1B and 1L) with regulatory (promoter) function (10). Regulation of TNAP gene expression is complex and far from being fully understood. However, transcription factors Sp1 and Sp3, retinoic acid (activated form of vitamin A), progestin, granulocyte colony stimulating factor and 1.25-dihydroxyvitamin D have been reported to regulate TNAP gene expression either by increasing transcriptional activity or by increasing TNAP mRNA stability (19,20). Mutations of TNAP gene cause hypophosphatasia, a rare inherited systemic bone disease that is characterized by bone hypomineralization (12,21). As of end of 2013, 275 mutations in the TNAP gene have been reported to cause hypophosphatasia (11).
Physiological functions of AP in tissues other than bone remain largely unknown. The participation of bone isoenzyme of AP in the skeletal mineralization—a hypothesis first proposed by Robison (3)—is already confirmed and is the best known AP physiological action. The role of AP in bone mineralization has been verified, particularly after recognition of an inborn error of metabolism involving TNAP which was associated with defective bone mineralization (22). Although, TNAP has a broad specificity and may cleave phosphate from multiple substrates, natural substrates are pyrophosphate, pyridoxal 5’-phosphate (PLP) and perhaps, phosphoethanolamine (23,24). Hydrolysis of pyrophosphate to produce two inorganic phosphates by the enzyme is important for bone mineralization for at least two reasons: first it increases the availability of phosphate which afterwards is incorporated in the structure of hydroxyapatite together with Ca2+; and second it decreases the concentration of pyrophosphate, a potent inhibitor of mineralization process (22). Pyridoxal-5’-phosphate (activated vitamin B6)—a co-enzyme that is required for >150 enzymatic reactions (25)—is also a physiological substrate for TNAP in leukocytes (26). Although, physiological consequences of TNAP action on pyridoxal-5’-phosphate remain largely unknown, in adults, common variants of TNAP gene influence plasma concentrations of this co-enzyme (27). Abnormal metabolism of pyridoxal-5’-phosphate may also explain epileptic seizures that experience some patients with hypophosphatasia (1). Other hypothetical but less known physiological AP actions have been reviewed (1,12,21,28). Lipopolysaccharide—a constituent of the cell wall of gram-negative bacteria—is also a substrate of intestinal AP. It is suggested that dephosphorilation of lipopolysaccharide by intestinal AP reduces its load and contributes to microbiome homeostasis in the intestinal tract (29).
Isoelectric focusing technique has detected more than 17 different AP isoforms in circulation (30). AP activity in serum originates mostly from liver and bone in similar proportions. Intestinal AP contributes to approximately 10% of overall AP activity (31). Circulating (soluble) AP is physiologically inactive (21). Placental type is expressed only during the last semester of pregnancy (32). After fatty meal, AP of intestinal origin is elevated in some individuals but it makes a small contribution (less than 20%) (33). Mechanism of AP release from tissues in circulation is unknown, but C or D phosphatidases, detergents, cell turnover, proteolysis, lipolysis or membrane fragmentation have been proposed (21). The AP activity in plasma ranges between 40 and 130 U/L in healthy men and between 35 and 105 U/L in healthy women (measured by a standardized, colorimetric enzyme-assay according to the International Federation of Clinical Chemistry and Laboratory Medicine method) (31). Measurement of circulating AP activity is extensively used for the diagnosis of bone and liver disease. Both elevation (more common) and decrease of circulating AP activity have clinical utility (28,34).
Even though, measurement of AP is not used for the diagnosis of cardiovascular disease (CVD), emerging evidence suggests that elevated AP activity indicates an increased risk for CVD and total or CVD-related mortality. This review aimed to summarize the existing knowledge linking AP with CVD.
AP and CVD and mortality
Epidemiological evidence
Epidemiological evidence gathered from population-based, longitudinal studies suggests an association between elevated AP levels and CVD or mortality. Tonelli et al. (35) analyzed the association between AP and cardiovascular outcomes in survivors of an acute myocardial infarction [the Cholesterol And Recurrent Events (CARE) study; n=4,115] and in a general population sample [the Third National Health and Nutrition Examination Survey (NHANES III); n=14,716 adults collected between 1988–1994 and followed up for a mean of 12 years]. The CARE study findings are discussed later in this review. In the NHANES III sample there was a graded association between AP and all-cause mortality [mortality rates, 4.7%, 8.2% and 13.0% in the 1st, 2nd and 3rd AP tertiles; adjusted hazard ratio (HR) =1.27, 95% confidence interval (CI): 1.06 to 1.52, P=0.01 for 3rd vs. 1st AP tertile]. With respect to cardiovascular mortality (mortality rates, 1.9%, 3.5% and 5.9% in the 1st, 2nd and 3rd AP tertiles), the association was significant in partially (age, sex and race-adjusted) adjusted analysis [HR =1.61 (1.24–2.09) for 3rd vs. 1st AP tertile] but it was attenuated after full adjustment [HR =1.27 (0.98–1.65), P=0.07]. In the NHANES III analysis, the strength of association did not differ according to presence of chronic kidney disease, proteinuria, smoking status, body mass index (dichotomized at 30 kg/m2), diabetes or C-reactive protein (CRP; dichotomized at 3 mg/L). The findings remained consistent in analyses restricted to participants without pre-existing coronary heart disease (CHD) or when participants were categorized according to AP quintiles. Of note, cubic spline regression showed a linear relationship between higher AP levels and the risk of all-cause mortality. The association between AP and mortality was stronger in the presence of elevated phosphate levels (35). Wannamethee et al. (36) assessed the association of AP with CVD outcomes and mortality in a sample of 3381 men, 60 to 79 years of age recruited in the British Regional Heart Study, all of whom were without pre-existing diagnosis of myocardial infarction or stroke. During a mean follow-up of 11 years, there were 317 CHD events [adjusted HR =1.15 (1.03–1.28)], 230 stroke events [adjusted HR =1.09 (0.96–1.25)], 345 CVD deaths [adjusted HR =1.07 (0.96–1.18)] and 605 CVD events [fatal CHD, nonfatal myocardial infarction, stroke or CVD death; adjusted HR =1.09 (1.01–1.18)], with all risk estimates calculated per standard deviation (SD) increase in log-AP. Overall there were 984 deaths [adjusted HR =1.09 (1.03–1.16) per each SD increase in log-AP]. Notably, all associations remained consistent after exclusion of subjects with chronic kidney disease. The study showed an association between AP and the risk of CHD and CVD events that was partially explainable by AP association with CVD risk factors and inflammation in elderly men free of myocardial infarction or stroke (at entry). The association between AP and total mortality was independent of inflammation or chronic kidney disease.
Other studies offer additional evidence for an association between elevated AP and increased risk of CVD or related outcomes. Abramowitz et al. (37) tested the association of AP (or phosphate) with the risk of mortality or hospitalization in a retrospective cohort study of 10,743 outpatients with preserved renal function (estimated glomerular filtration rate of >60 mL/min/1.73 m2) recruited between 2000 and 2002 and followed up to a median of 6.8 years. The mean age was 51 years and women comprised 64% of the cohort. Overall 949 patients died during the follow-up. There was a progressive increase in the risk of mortality from AP quartiles 1 (AP ≤66 U/L) to 4 (AP ≥104 U/L; P for trend <0.001). In the risk assessment analysis, the unadjusted and fully adjusted HR for the association of AP with mortality were 2.05 (1.70–2.48) and 1.65 (1.36–2.01), respectively (for AP quartile 4 vs. quartile 1). AP was also associated with the risk of all-cause [adjusted HR =1.14 (1.03–1.25)], CVD [adjusted HR =1.28 (1.10–1.48)], infection [adjusted HR=1.53 (1.28–1.84)] or fracture-related [adjusted HR =1.63 (1.03–2.58)] hospitalization (with all risk estimates calculated for 4th vs. 1st AP quartile). Filipowicz et al. (38) assessed the association of skeletal and non-skeletal AP with CRP or mortality in a sample of 10,707 adults from the 1999–2004 NHANES participants. The total serum AP showed a moderate correlation with skeletal AP (Pearson r=0.74, P<0.001) assuming that 55% of variation in serum AP could be due to variation in skeletal AP. There were 715 deaths over a follow-up of 54,089 person-years. Total AP was associated with the risk of elevated CRP [adjusted odds ratio (OR) =1.96 (1.74–2.22)] and mortality [HR =1.28 (1.08–1.52)]. However, there were differences with respect to the association of skeletal and non-skeletal AP with CRP or mortality. Skeletal AP was not associated with CRP or mortality in subjects with or without chronic kidney disease. Conversely, non-skeletal AP was associated with elevated CRP in subjects without chronic kidney disease [OR =4.51 (3.80–5.35)] and those with chronic kidney disease [OR =5.98 (3.40–10.51)]. Of note, non-skeletal fraction was associated with the risk of mortality in subjects without chronic kidney disease [HR =1.96 (1.37–2.80)] but not in those with chronic kidney disease [HR =0.92 (0.51–1.67)] with all risk estimates calculated per doubling of serum skeletal AP (P for interaction =0.03). The study showed that non-skeletal sources of AP elevation, but not bone disease, may explain the association between total AP and increased risk of mortality or inflammation. Koehler et al. (39) assessed the association of liver enzymes with all-cause and cause-specific mortality in 5,186 participants (61.6% women) aged 55 years or older, embedded in the Rotterdam Study. During a follow-up of up to 19.5 years (median: 14 years), 2,997 participants (57.8%) died. Of all the deaths, 672 were of CVD origin and 703 were due to cancer. AP was associated with all-cause [adjusted HR =1.51 (1.28–1.77)], CVD [adjusted HR =1.43 (1.01–2.04)] and cancer-related [adjusted HR =1.57 (1.12–2.20)] mortality, with all risk estimates calculated for AP>95th percentile (124 U/L in women and 119 U/L in men) vs. <25th percentile (61 U/L in men and 64 U/L in women). Findings remained consistent when hemoglobin and phosphate values were added into the multivariable models. The study showed that an elevated AP level is associated with the risk of mortality from cancer and CVD in subjects >55 years of age. Fulks et al. (40) investigated the relationship between liver enzymes (including AP) and all-cause mortality in life insurance applicants (n=1,905,664). There were 50,174 deaths over a median follow-up of 12 years. Using the risk of the middle 50% of the population (25th to 74th percentiles) as reference, the relative risk (RR) of mortality increased significantly and was linear from very low to relatively high values of AP. Recently, Kunutsor et al. (41) assessed the association of AP with CVD risk in 6,974 participants of the Prevention of Renal and Vascular End-stage Disease (PREVEND) study—an observational, general population-based cohort study. AP correlated with several CVD risk factors, notably age and CRP. Seven hundred and thirty seven participants developed CVD over a median follow-up of 10.5 years. AP showed a “J-shaped” relationship with the risk for developing CVD. After adjustment for conventional CVD risk factors, the HR for an association between AP and CVD was 1.34 (1.14–1.56) for 5th vs. pooled 1st–4th AP quintiles. The association persisted after adjustment for additional confounders but was attenuated after adjustment for CRP [HR =1.24 (1.05–1.45)]. Of note, addition of AP in multivariable risk prediction models containing established CVD risk factors, did not improve the risk prediction for incident CVD by these models, as judged by the degree of change of C statistic or net reclassification improvement. In a sample of 1,054 participants aged 65 years or over (49% women) from the British National Diet and Nutrition Survey, the association between bone-related markers (including AP) and subsequent mortality was analyzed. Over a 13 to 14 years of follow-up, AP was associated with mortality in women [age-adjusted HR =1.08 (1.01–1.16)] but not in men [age-adjusted HR =1.06 (0.89–1.26)]. However, after full adjustment, the association was attenuated to below the significance level (42).
AP has been shown to predict mortality in patients with chronic kidney disease and dialysis. A study by Regidor et al. (43) that included a 3-year cohort of 73,960 hemodialysis patients showed that an AP level ≥120 U/L was associated with a 25% increase in the adjusted risk for all-cause mortality [HR =1.25 (1.21–1.29)]. A rise in AP by 10 U/L over the first 6 months was incrementally associated with higher risk of death in the subsequent 2.5 years. Three-year cardiovascular mortality was also increased with the increase of AP level. There was a somewhat linear relationship between AP and mortality. Recently, bone AP has been proposed as a potential target of drug therapy to improve cardiovascular outcomes in patients with chronic kidney disease (44).
Three recent meta-analyses have summarized epidemiological evidence on the association between AP and the risk of CVD or mortality (45-47). A meta-analysis by Kunutsor et al. (45) that included four prospective cohort studies with 33,727 participants and 2,097 cases reported a 8% increase [RR =1.08 (1.03–1.14)] in the risk for CVD for each SD increase in AP level. Another meta-analysis of 4 prospective studies reported a 38% increase in the risk for all-cause mortality [RR =1.38 (1.17–1.63)] in individuals in the top third vs. those in the bottom third of AP level (45). Finally, a recent meta-analysis by Li et al. (47) that included only studies of subjects with preserved renal function reported a 2% increase in the risk for CVD death [RR =1.02 (1.01–1.04)] and a risk of 57% [RR=1.57 (1.27–1.95)] for all-cause mortality for high vs. low AP group. The AP-mortality relationship was non-linear.
In conclusion, epidemiological evidence is strong for an association between elevated AP activity and the risk of all-cause mortality in general population. Although several studies have reported an increased risk for incident CVD in subjects with elevated AP level, evidence is weaker for an association between elevated AP and the risk for incident CVD or CVD-related mortality. Evidence remains inconclusive with respect to gender-related disparities in the association between AP and the risk for mortality or CVD. The association between elevated AP and the risk of atherosclerosis, CHD or acute coronary events such as acute myocardial infarction remains partially investigated. An elevated AP level appears to be associated with reduced survival in patients with chronic kidney disease.
AP and stroke
Epidemiological evidence suggests an association between elevated AP and the risk of stroke. In the British Regional Heart Study, elevated AP was associated with the increased risk of stroke over a mean follow-up of 12 years in men without myocardial infarction or stroke at baseline. In subjects with AP in the 1st, 2nd, 3rd and 4th AP quartiles, the stroke rates per 1,000 person-years were 5.5, 6.9, 6.5 and 9.5, respectively. The association was significant in age-adjusted analysis [HR =1.15 (1.03–1.29)] but it was attenuated after full adjustment [HR =1.09 (0.96–1.25)], with both HRs calculated per SD increase in log-AP (36). Shimizu et al. (48) assessed the association of AP with the risk of stroke in a cohort of 10 754 Japanese subjects (4,098 men and 6,656 women; aged 40–69 years) recruited in the Circulatory Risk in Communities Study (CIRCS) study. During a 16-year follow-up, there were 264 strokes (164 ischemic; 69 hemorrhagic) in men and 225 strokes (118 ischemic; 89 hemorrhagic) in women.
There was a U-shaped relationship between AP level and incidence of stroke in men and women which for the most part was confined to non-drinkers. The fully adjusted HR for association of AP with total strokes was 1.79 (1.20–2.68) in men and 1.29 (0.85–1.96) in women. According to drinking status the fully adjusted HRs in non-drinkers were 5.90 (2.01–17.33) in men and 1.43 (0.92–2.22) in women; in drinkers, the fully adjusted HRs were 1.32 (0.83–2.11) and 0.31 (0.06–1.74), in men and women, respectively, with all HR calculated for AP 5th quintile vs. 3rd quintile (used as reference in the setting of U-shaped relationship). In non-drinkers, AP was associated with the risk of ischemic stroke in men and hemorrhagic stroke in women. Lower AP levels were associated with higher risk of hemorrhagic stroke in both genders. Although, alcohol consumption is known to be associated with the risk of stroke (both ischemic and hemorrhagic) (49), the CIRCS study showed an association between AP and stroke only in non-drinkers (48). In a cohort of 3,916 stroke-free participants ≥55 years of age from the Rotterdam Study, AP was associated with the risk of hemorrhagic stroke [55 hemorrhagic strokes over 50,375 person years of follow-up: adjusted HR=1.30 (1.02–1.68) per SD increment of AP level]. The association was stronger in excessive alcohol drinkers than in non-excessive alcohol drinkers (50).
Several studies have shown that increased AP is associated with poor outcomes after stroke (51,52). A prospective study of 2,029 stroke patients, followed up for a mean of 923 days showed a significant association between baseline AP and all-cause death with adjusted HR for 3rd, 4th and 5th AP quintiles of 1.67 (1.12–2.49), 1.79 (1.20–2.67) and 2.83 (1.95–4.10) compared with 1st AP quintile. The associations were significant for ischemic and hemorrhagic strokes. The risk for vascular death was also higher in patients with elevated AP level with HR for 4th and 5th AP quintiles (vs. the 1st quintile) of 1.81 (1.14–2.86) and 2.78 (1.87–4.15), respectively (51). In another more recent study of 1,034 patients with first-ever acute cerebral infarction, AP levels were associated with a poor functional outcome [OR =1.25 (1.04–1.50) for each SD increment of AP] but not with cerebral atherosclerosis (52). In other studies, AP has been associated with cerebral small vessel disease—a marker of silent brain (lacunar) infarction (53), multi-cerebral microbleeds in patients with acute ischemic stroke (54) or transformation of ischemic to hemorrhagic stroke in patients with atrial fibrillation (55). In another recent study of 1,082 neurologically healthy subjects undergoing brain magnetic resonance imaging, elevated AP was independently associated with white matter hyperintensities and cerebral infarct but not with microbleeds (56). Although such conditions help to explain an elevated risk of stroke in subjects with elevated AP and may impact unfavourably on prognosis of patients after stroke, causality remains unproven.
In aggregate, there is limited (particularly compared to evidence linking AP with the risk of all-cause mortality) but strongly suggestive epidemiological evidence for an association between AP and increased risk of stroke. Evidence available also suggests that subjects with elevated AP may be at higher risk of a poor functional outcome or mortality following a stroke event. Gender-related disparities in the association between AP and the risk of stroke and confounding effect of drinking on the AP-stroke association require further study.
AP and mortality in patients with CHD
Patients with known CHD are at higher risk of mortality primarily due to CVD causes and biomarkers are valuable tools for risk stratification in these patients. A number of studies have investigated the prognostic value of AP in various subsets of patients with CHD. The association between AP and subsequent outcomes was assessed in 4,115 subjects with a previous myocardial infarction in the setting of CARE study (35). A graded, independent association was noted between AP tertiles and the risk for all-cause mortality over a median follow-up of 58.9 months [mortality rates 7%, 9% and 11% in the 1st, 2nd, and 3rd AP tertiles: fully adjusted HR =1.43 (1.09-1.89)]. However, there was no independent association between AP and CHD death [adjusted HR =1.13 (0.78–1.63)] or nonfatal myocardial infarction [adjusted HR=1.23 (0.94–1.62)]. For the association between AP and the composite end point of fatal CHD, nonfatal myocardial infarction, symptomatic heart failure or stroke, the significance was borderline [adjusted HR =1.21 (0.98–1.46). P=0.05] with all risk estimates calculated for highest vs. lowest AP tertile. Thus the CARE study showed an independent association between AP and all-cause mortality but not an association with CHD-related death or acute coronary events (35). Park et al. (57) assessed the association of AP with prognosis of patients with CHD who underwent percutaneous coronary intervention with drug-eluting stent implantation in 1,636 patients without known liver disease or cancer (at baseline) over a median follow-up of 762 days. The rates of all-cause and cardiac mortality in the 1st, 2nd and 3rd AP tertiles were 3.1%, 5.5% and 13.2% and 1.8%, 2.5% and 6.6% respectively: adjusted HR =4.21 (2.03–8.71) for all-cause mortality and adjusted HR =3.92 (1.37–11.20) for cardiac mortality. AP also was associated with the risk of nonfatal myocardial infarction [adjusted HR =1.98 (0.91–4.29)], and stent thrombosis [adjusted HR =2.73 (1.33–5.61)], with all HR calculated for highest vs. lowest AP tertile. Of note, AP was significantly associated with the presence of angiographic coronary calcium (P=0.046). Our group assessed the association between AP and 3-year outcome in 5,540 patients with angiography-proven coronary artery disease treated with percutaneous coronary intervention (58). The 3-year mortality rates according to AP tertiles were 7.2%, 8.1% and 11.8% in the 1st, 2nd and 3rd AP tertiles with HR =1.33 (1.19–1.50) calculated per each tertile increment. After adjustment, the association between AP and all-cause mortality remained significant [adjusted HR =1.33 (1.18–1.51), calculated per unit increment in log-AP]. Adding AP in multivariable models alongside cardiovascular risk factors and CRP improved the risk prediction for all-cause mortality as assessed by C-statistic changes [C-statistic without AP, 0.820 (0.797–0.843) vs. C-statistic 0.825 (0.804–0.849) after AP inclusion; P<0.001]. The association with cardiac mortality was also significant [adjusted HR =1.40 (1.20–1.62) per unit log-AP]. The study did not find an association between AP and coronary calcium or the risk of acute coronary events (nonfatal myocardial infarction or stent thrombosis) or stroke. Moreover, the association between AP and all-cause mortality was stronger in subjects of younger age (<65 years). Of note, chronic statin therapy was associated with reduced levels of circulating AP (58).
Few studies have investigated the association between AP and prognosis in patients with acute coronary syndromes and ST-segment elevation myocardial infarction (STEMI). In a recent study by our group, the 3-year prognostic value of AP was tested in 2,134 patients with acute coronary syndromes after percutaneous coronary intervention (59). Using a cut-off of 98 U/L (defined by receiver operating characteristic curve analysis), mortality rates were 19.5% and 9.3%, in patients with AP >98 and ≤98 U/L, respectively [adjusted HR =1.37 (1.10–1.70) for each unit increment in log-AP]. AP was also associated with the risk of cardiac mortality [mortality rates 14.3% and 6.0% in patients with AP >98 and ≤98 U/L, respectively (adjusted HR =1.32 (1.02–1.70), for each unit increment in log-AP]. The C-statistic was improved by AP inclusion in multivariable models of all-cause mortality [0.836 (0.807–0.866) before vs. 0.842 (0.814–0.874) after AP inclusion; P=0.045] but not in the models of cardiac mortality [0.854 (0.823–0.884) before and 0.857 (0.824–0.889) after AP inclusion; P=0.385]. The study found a stronger association between AP and all-cause mortality in patients of younger age (<65 years) and those with preserved renal function (P for interaction=0.006 and 0.038, respectively). With respect to cardiac mortality the association was stronger in patients of younger age (<65 years; P for interaction =0.028) (59). A recent study that assessed the prognostic value of liver enzymes in 847 patients in acute phase of STEMI showed that AP was the strongest correlate of in-hospital major adverse cardiovascular events [OR =1.02 (1.01–1.03)] (60). Another study of diabetic patients presenting with acute myocardial infarction showed that elevated AP was associated with reduced subsequent 5-year survival (61). A recent study in 1178 Korean STEMI patients assessed the association of AP measured at presentation and major adverse cardiovascular events—a composite of all-cause death, non-fatal myocardial infarction, non-fatal stroke, and ischemia-driven revascularization over a median follow-up of 25 months. The AP level was below the upper limit of normal in about 95% of patients. There was a progressive increase in the incidence of adverse events from 8.7% to 11.7% and 15.7% across AP tertiles (<64, 65–82 and >83 U/L, respectively) with adjusted HR =1.69 (1.01–2.81) for 2nd vs. 1st AP tertile and HR =2.46 (1.48–4.09) for 3rd vs. 1st AP tertile (62).
In summary, available evidence suggests that elevated AP is associated with the increased risk of death in patients with stable CHD or acute coronary syndromes. However, several aspects of this association remain unclear. In the absence of comparative studies in patients with proven CHD and subjects without CHD, the question whether the presence of CHD strengthens the association with mortality remains unanswered. Although patients with CHD are expected to die mostly from cardiac causes, the association of AP with cardiac mortality seems to be weaker than the association of AP with all-cause mortality and the reasons for this remain unknown. The association between AP, coronary calcium and acute coronary events remains controversial. Finally, the impact of percutaneous coronary intervention or current cardiovascular drugs (particularly statins) on the association between AP and outcome in patients with CHD is plausible but needs further study.
AP and congestive heart failure
Abnormal liver tests, including abnormal AP values are common in patients with congestive heart failure (63-65) and caused by liver congestion and injury in the setting of this syndrome (66). Very high AP levels in patients with congestive heart failure have been described (67,68). In the CARE study, a progressive increase in the frequency of symptomatic congestive heart failure with the increase in AP activity was observed [adjusted HR=1.38 (1.01–1.88), for highest vs. lowest AP tertile] (35). Several studies have suggested that elevated AP is associated with poor prognosis in patients with congestive heart failure. In an analysis from the SURVIVE (Survival of Patients With Acute Heart Failure in Need of Intravenous Inotropic Support) trial that included 1,134 patients, 11% of patients had abnormal AP values (64). Of note, patients with abnormal AP had marked signs of congestion, elevated right-sided filling pressures and increased 6-month mortality (64). Poelzl et al. (69) assessed the association of liver function tests in 1,032 patients with stable congestive heart failure. In this study cholestatic enzymes, but not amino transferases were associated with severity of disease. Over a median follow-up of 36 months, death or heart transplantation (primary end point) was recorded in 339 patients. Total bilirubin, AP and gamma-glutamyl transferease were associated with the outcome in bivariate analysis. In multivariable analysis, AP [adjusted HR =1.52 (1.09–2.12)] and gamma-glutamyl transferase [adjusted HR =1.22 (1.06–1.41) with both HR calculated per log units of the biomarkers] remained independently associated with the risk of primary endpoint (69). In other studies, however, the association between elevated AP and prognosis was attenuated after adjustment in multivariable analysis (65,70). A recent retrospective analysis of 972 patients showed that elevated AP was associated with the risk of worsening of renal function in the course of acute decompensated heart failure [adjusted OR =1.69 (1.02–2.79) for 2nd vs. 1st AP tertile and adjusted OR=1.95 (1.20–3.21) for 3rd vs. 1st AP tertile] (71). These studies almost unanimously showed that liver function tests including AP are frequently abnormal in patients with congestive heart failure and they signify a poor prognosis in these patients.
AP and cardio-metabolic risk factors
Many studies have shown an association between AP and several cardio-metabolic risk factors. In the British Regional Heart Study, AP was significantly associated with older age, smoking, physical inactivity, elevated systolic blood pressure, low-density lipoprotein cholesterol, CRP, reduced renal function, von Willebrand factor and reduced lung function (36). In the CARE study that included subjects with definite diagnosis of previous infarction, AP was strongly associated with older age, sex (higher proportion of women in top AP tertile), alcohol consumption, smoking, proteinuria, systolic blood pressure, lower high-density lipoprotein, fasting glucose and diabetes (35). In the NHANES III data set, AP was strongly associated with older age, male sex, smoking, alcohol consumption, body mass index, diabetes, albuminuria, lower high-density lipoprotein level, higher systolic blood pressure, lower 25(OH)-vitamin D and higher CRP-level (35). In the Rotterdam Study, higher AP levels were associated with older age, female sex, diabetes, current smoking and arterial hypertension as well as lower alcohol intake and elevated cholesterol (39). A particularly strong correlation between AP and elevated CRP has been reported (41,72-74). It has been suggested that AP represents an acute phase reactant and a marker of inflammation of hepatic origin (72) or that the association between AP and increased CVD risk may be mediated by inflammation (41). A retrospective analysis of 15,234 adult participants in the NHANES III study showed a progressive increase in the frequency of metabolic syndrome with the increase in AP level (frequency 14% among subjects in lowest AP quartile and 41% among subjects in highest AP quartile). In subjects with AP in 2nd, 3rd and 4th AP quartiles, the adjusted ORs for the risk of metabolic syndrome were 1.41, 1.66 and 1.75 compared with AP 1st quartile (reference). However, the association of AP with mortality remained significant after adjusting for demographic factors [HR =1.52 (1.35–1.72)] or demographic factors plus metabolic syndrome [HR =1.37 (1.21–1.56)]. The association was stronger in a subgroup of subjects without any component of metabolic syndrome [HR =1.83 (1.36–2.46)] (75). A large Chinese case-control study that included 6,268 subjects with metabolic syndrome and 6,330 matched healthy controls showed that the risk of metabolic syndrome increased progressively with the increase in AP activity with OR=1.43 (1.28–169); OR=1.87 (1.68–2.08) and OR=2.80 (2.52–3.10) for the 2nd, 3rd and 4th AP quartiles vs. the 1st quartile. The risk of metabolic syndrome was increased by 9% [adjusted OR=1.09 (1.08–1.10)] for each 5 units increase in the AP activity (76). Based on these studies, the evidence is clear that elevated AP levels signify a worse cardio-metabolic risk profile and the close association between AP and CVD risk factors may account at least in part for the association between AP and the risk of CVD or mortality.
Putative mechanisms of the association between AP and CVD risk and mortality
Outside clinically overt bone or liver disease (both conditions may affect CVD risk and survival), mechanisms for the association of AP with CVD risk or mortality remain incompletely investigated. Nevertheless, a number of potential mechanisms may be mentioned.
First, as stated above, elevated AP level is associated with a plethora of cardio-metabolic risk factors. This association has at least two implications: firstly, the association implies that a part of the risk associated with elevated AP levels is mediated by cardio-metabolic risk factors; secondly, the association raises concerns with respect to whether AP has a direct role in the pathophysiology of CVD and the risk of mortality or it is simply an epiphenomenon of CVD risk factors which tend to cluster in subjects with elevated AP level.
Second, AP is strongly associated with markers of systemic inflammation, in particular CRP. According to current paradigm, inflammation plays a crucial role in all stages of atherosclerosis including atherosclerotic plaque initiation, progression and transition from stable to unstable plaques with subsequent clinical events (77).
Third, elevated AP has been reported to reflect low levels of vitamin D in population-based studies (35) and patients with chronic kidney disease on dialysis (78). A prospective study by Giovannucci et al. (79) showed that vitamin D deficiency was associated with the risk of myocardial infarction in a graded manner and independent of CVD risk factors in men. Other studies have shown that up to 90% of patients presenting with ST-segment elevation myocardial infarction had low levels of vitamin D (80) or that vitamin D deficiency was associated with 1-year mortality in patients presenting with acute coronary syndromes (81). In fact, vitamin D deficiency is an emerging CVD risk factor and potentially relevant mechanisms explaining the association between vitamin D deficiency and cardiovascular risk and mortality may include the association of low vitamin D levels with smooth muscle cell proliferation, endothelial dysfunction, vascular inflammation, vascular calcification, increased activity of renin-angiotensin system and atherosclerosis (82-84). Despite this evidence a recent randomized, double-blinded, placebo-controlled study found no effect of vitamin D supplementation for 8 weeks on bone turnover markers (including bone AP) in 197 hypertensive patients (85).
Fourth, elevated AP may be associated with liver disease, primarily non-alcoholic fatty liver disease—a highly prevalent morbid condition and a common cause of elevated liver enzymes (86). Multiple lines of evidence suggest that non-alcoholic fatty liver disease is closely associated and shares many features with metabolic syndrome and other factors predisposing for coronary atherosclerosis and CVD (87). CVD remains a common cause of death in patients with non-alcoholic fatty liver disease and patients with this condition may benefit from CVD risk assessment (88). However, a recent analysis from the PREVEND study involving 6,340 participants aged 28–75 years without pre-existing CVD found no association between non-alcoholic fatty liver disease and the risk of CVD, once the association was adjusted for established CVD risk factors and other potential confounders (89).
Although important for understanding the association between AP and the risk of CVD or mortality, none of the above-cited mechanisms points out to a direct role of AP in the pathophysiology of CVD or CHD. Demonstration of the involvement of AP in vascular calcification (90) has led to the hypothesis that AP mediates elevated CVD risk and a deleterious prognostic impact through vascular calcification. Mechanisms of vascular calcification have been recently reviewed (91). Several lines of evidence seem to support such a role. AP activity is crucial for formation of hydroxyapatite—a crucial molecule in the endochondral calcification or vascular calcification when AP is expressed in osteoblast-type cells originating from phenotypic transformation of smooth muscle cells (92). AP stimulates vascular smooth muscle cell trans-differentiation into chondrocyte-like cells (93), a pivotal step in vascular calcification. Up-regulation of AP expression in vessels with medial calcification (94) or advanced calcified atherosclerotic lesions (95), hydrolysis of pyrophosphate—an inhibitor of calcification—by up-regulated AP (96) and inhibition of vascular calcification by AP inhibitors (97) appear to offer evidence in favor of AP involvement in vascular calcification. An experimental mouse model involving overexpression of human TNAP in vascular smooth muscle cells demonstrated extensive medial vascular calcification early in life, in the absence of systemic changes in calcium, phosphate, or renal function (98). Medial calcification leads to vascular stiffness and consequently to increased pulse pressure (99) and left ventricular hypertrophy (100)—factors associated with the risk of mortality in patients with CHD. Although, the association of vascular calcification with atherosclerosis remains poorly understood (92), medial artery calcification is associated with CHD and increased risk of mortality in patients with chronic kidney disease (101) and diabetes (102). Coronary calcium also predicts mortality in community-living individuals (103). Whether, atherosclerotic plaque calcification contributes to plaque instability remains controversial (92). Some authors have suggested that presence of calcium destabilizes the plaque either because of a physical stress exerted by calcified nodules (104) or propensity of plaques to rupture at areas of interface between high and low density tissue (105). Spotty calcification has been suggested to predispose for atherosclerotic plaque rupture, as well (106). Putative mechanisms of AP association with CVD are shown in Figure 1.
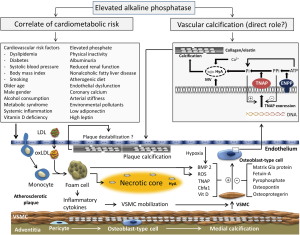
A number of studies have suggested an association of AP with several conditions that may increase CVD risk or mortality. Thus AP has been shown to be associated with the extent of coronary artery disease (107), lack of collateral circulation (108), contrast-induced nephropathy in patients with acute coronary syndromes (109), arterial stiffness after renal transplantation (110), left ventricular hypertrophy in mice (111), endothelium-dependent vasodilation and endothelial dysfunction in hypertensive patients (112), adipogenesis (113), atherogenic diet (33), phosphate metabolism (36), low adiponectin (114), high leptin (115), fibroblast growth factor-23 (116) or environmental pollutants (117). Although these conditions may increase the risk of CVD or have a negative impact on prognosis, causality relationship between them and AP remains unproven.
Conclusions and perspective
Population-based longitudinal studies suggest an association between AP and increased risk of incident CVD or all-cause mortality. Clinical studies involving patients with pre-existing CHD also suggest an association between AP and mortality in these patients. The association between AP and increased risk of mortality seems to be stronger for all-cause mortality than for cardiac mortality. Experimental studies strongly suggest an involvement of AP in vascular calcification and a direct role of the enzyme in the pathophysiology of CVD. There is no consistent evidence with respect to association of AP with extent of CHD, angiographic coronary calcification or acute coronary events such as acute myocardial infarction. The association between AP and other CVD such as arterial hypertension or cardiac arrhythmias, primarily atrial fibrillation remains unexplored. AP is associated with cardiometabolic risk factors including conventional CVD risk factors, metabolic syndrome, systemic inflammation, vitamin D deficiency and several morbid conditions that may signify a poor CVD risk profile and increased risk for mortality. The intricate relationship between AP and cardio-metabolic risk factors and failure to improve risk prediction for incident CHD (41) or cardiac mortality in patients with acute coronary syndromes (59) by the enzyme seem to weaken arguments for a causal relationship between AP and CVD. Further explorations of the molecular mechanisms of the involvement of AP in the pathophysiology of CVD and the use of the enzyme as a therapeutic target to reduce CVD risk remain to be elucidated in future studies.
Acknowledgments
Funding: None.
Footnote
Conflicts of Interest: The author has completed the ICMJE uniform disclosure form (available at http://dx.doi.org/10.21037/jlpm.2017.10.02). The author has no conflicts of interest to declare.
Ethical Statement: The author is accountable for all aspects of the work in ensuring that questions related to the accuracy or integrity of any part of the work are appropriately investigated and resolved.
Open Access Statement: This is an Open Access article distributed in accordance with the Creative Commons Attribution-NonCommercial-NoDerivs 4.0 International License (CC BY-NC-ND 4.0), which permits the non-commercial replication and distribution of the article with the strict proviso that no changes or edits are made and the original work is properly cited (including links to both the formal publication through the relevant DOI and the license). See: https://creativecommons.org/licenses/by-nc-nd/4.0/.
References
- Millan JL. Alkaline Phosphatases: Structure, substrate specificity and functional relatedness to other members of a large superfamily of enzymes. Purinergic Signal 2006;2:335-41. [Crossref] [PubMed]
- Suzuki U, Yoshimura K, Takaishi M. Uber ein enzyme “phytase” das anhydro-oxy-methylen-diphosphorsaure spaltet. Bull Coll Agric Tokyo Imp Univ 1907;7:503-12.
- Robison R. The possible significance of hexosephosphoric esters in ossification. Biochem J 1923;17:286-93. [Crossref] [PubMed]
- Robison R, Soames KM. The possible significance of hexosephosphoric esters in ossification. part III: The action of the bone enzyme on the organic phosphorus compounds in blood. Biochem J 1924;18:740-54. [Crossref] [PubMed]
- Kay HD, Robison R. The possible significance of hexosephosphoric esters in ossification. part III: The action of the bone enzyme on the organic phosphorus compounds in blood. Biochem J 1924;18:755-64. [Crossref] [PubMed]
- Martland M, Robison R. Possible significance of hexosephosphoric esters in ossification: Part VI. phosphoric esters in blood-plasma. Biochem J 1926;20:847-55. [Crossref] [PubMed]
- Siller AF, Whyte MP. Alkaline Phosphatase: Discovery and Naming of Our Favorite Enzyme. J Bone Miner Res 2017; [Epub ahead of print]. [Crossref] [PubMed]
- Mornet E, Stura E, Lia-Baldini AS, et al. Structural evidence for a functional role of human tissue nonspecific alkaline phosphatase in bone mineralization. J Biol Chem 2001;276:31171-8. [Crossref] [PubMed]
- Weiss MJ, Cole DE, Ray K, et al. A missense mutation in the human liver/bone/kidney alkaline phosphatase gene causing a lethal form of hypophosphatasia. Proc Natl Acad Sci U S A 1988;85:7666-9. [Crossref] [PubMed]
- Matsuura S, Kishi F, Kajii T. Characterization of a 5'-flanking region of the human liver/bone/kidney alkaline phosphatase gene: two kinds of mRNA from a single gene. Biochem Biophys Res Commun 1990;168:993-1000. [Crossref] [PubMed]
- Silvent J, Gasse B, Mornet E, et al. Molecular evolution of the tissue-nonspecific alkaline phosphatase allows prediction and validation of missense mutations responsible for hypophosphatasia. J Biol Chem 2014;289:24168-79. [Crossref] [PubMed]
- Orimo H. The mechanism of mineralization and the role of alkaline phosphatase in health and disease. J Nippon Med Sch 2010;77:4-12. [Crossref] [PubMed]
- Weiss MJ, Henthorn PS, Lafferty MA, et al. Isolation and characterization of a cDNA encoding a human liver/bone/kidney-type alkaline phosphatase. Proc Natl Acad Sci U S A 1986;83:7182-6. [Crossref] [PubMed]
- Nosjean O, Koyama I, Goseki M, et al. Human tissue non-specific alkaline phosphatases: sugar-moiety-induced enzymic and antigenic modulations and genetic aspects. Biochem J 1997;321:297-303. [Crossref] [PubMed]
- Butterworth PJ. Alkaline phosphatase. Biochemistry of mammalian alkaline phosphatases. Cell Biochem Funct 1983;1:66-70. [Crossref] [PubMed]
- Jemmerson R, Low MG. Phosphatidylinositol anchor of HeLa cell alkaline phosphatase. Biochemistry 1987;26:5703-9. [Crossref] [PubMed]
- Kiledjian M, Kadesch T. Post-transcriptional regulation of the human liver/bone/kidney alkaline phosphatase gene. J Biol Chem 1991;266:4207-13. [PubMed]
- Riancho-Zarrabeitia L, Garcia-Unzueta M, Tenorio JA, et al. Clinical, biochemical and genetic spectrum of low alkaline phosphatase levels in adults. Eur J Intern Med 2016;29:40-5. [Crossref] [PubMed]
- Orimo H, Shimada T. Regulation of the human tissue-nonspecific alkaline phosphatase gene expression by all-trans-retinoic acid in SaOS-2 osteosarcoma cell line. Bone 2005;36:866-76. [Crossref] [PubMed]
- Orimo H, Shimada T. Posttranscriptional modulation of the human tissue-nonspecific alkaline phosphatase gene expression by 1,25-dihydroxyvitamin D-3 in MG-63 osteoblastic osteosarcoma cells. Nutrition Research 2006;26:227-34. [Crossref]
- Whyte MP. Physiological role of alkaline phosphatase explored in hypophosphatasia. Ann N Y Acad Sci 2010;1192:190-200. [Crossref] [PubMed]
- Millan JL, Whyte MP. Alkaline Phosphatase and Hypophosphatasia. Calcif Tissue Int 2016;98:398-416. [Crossref] [PubMed]
- Fedde KN, Whyte MP. Alkaline phosphatase (tissue-nonspecific isoenzyme) is a phosphoethanolamine and pyridoxal-5'-phosphate ectophosphatase: normal and hypophosphatasia fibroblast study. Am J Hum Genet 1990;47:767-75. [PubMed]
- Fedde KN, Blair L, Silverstein J, et al. Alkaline phosphatase knock-out mice recapitulate the metabolic and skeletal defects of infantile hypophosphatasia. J Bone Miner Res 1999;14:2015-26. [Crossref] [PubMed]
- Percudani R, Peracchi A. A genomic overview of pyridoxal-phosphate-dependent enzymes. EMBO Rep 2003;4:850-4. [Crossref] [PubMed]
- Wilson PD, Smith GP, Peters TJ. Pyridoxal 5'-phosphate: a possible physiological substrate for alkaline phosphatase in human neutrophils. Histochem J 1983;15:257-64. [Crossref] [PubMed]
- Carter TC, Pangilinan F, Molloy AM, et al. Common Variants at Putative Regulatory Sites of the Tissue Nonspecific Alkaline Phosphatase Gene Influence Circulating Pyridoxal 5'-Phosphate Concentration in Healthy Adults. J Nutr 2015;145:1386-93. [Crossref] [PubMed]
- Sharma U, Pal D, Prasad R. Alkaline phosphatase: an overview. Indian J Clin Biochem 2014;29:269-78. [Crossref] [PubMed]
- Fawley J, Gourlay DM. Intestinal alkaline phosphatase: a summary of its role in clinical disease. J Surg Res 2016;202:225-34. [Crossref] [PubMed]
- Wallace BH, Lott JA, Griffiths J, et al. Isoforms of alkaline phosphatase determined by isoelectric focusing in patients with chronic liver disorders. Eur J Clin Chem Clin Biochem 1996;34:711-20. [PubMed]
- Schumann G, Klauke R, Canalias F, et al. IFCC primary reference procedures for the measurement of catalytic activity concentrations of enzymes at 37 degrees C. Part 9: reference procedure for the measurement of catalytic concentration of alkaline phosphatase International Federation of Clinical Chemistry and Laboratory Medicine (IFCC) Scientific Division, Committee on Reference Systems of Enzymes (C-RSE) (1)). Clin Chem Lab Med 2011;49:1439-46. [Crossref] [PubMed]
- Okesina AB, Donaldson D, Lascelles PT, et al. Effect of gestational age on levels of serum alkaline phosphatase isoenzymes in healthy pregnant women. Int J Gynaecol Obstet 1995;48:25-9. [Crossref] [PubMed]
- Domar U, Karpe F, Hamsten A, et al. Human intestinal alkaline phosphatase--release to the blood is linked to lipid absorption, but removal from the blood is not linked to lipoprotein clearance. Eur J Clin Invest 1993;23:753-60. [Crossref] [PubMed]
- Schiele F, Vincent-Viry M, Fournier B, et al. Biological effects of eleven combined oral contraceptives on serum triglycerides, gamma-glutamyltransferase, alkaline phosphatase, bilirubin and other biochemical variables. Clin Chem Lab Med 1998;36:871-8. [Crossref] [PubMed]
- Tonelli M, Curhan G, Pfeffer M, et al. Relation between alkaline phosphatase, serum phosphate, and all-cause or cardiovascular mortality. Circulation 2009;120:1784-92. [Crossref] [PubMed]
- Wannamethee SG, Sattar N, Papcosta O, et al. Alkaline phosphatase, serum phosphate, and incident cardiovascular disease and total mortality in older men. Arterioscler Thromb Vasc Biol 2013;33:1070-6. [Crossref] [PubMed]
- Abramowitz M, Muntner P, Coco M, et al. Serum alkaline phosphatase and phosphate and risk of mortality and hospitalization. Clin J Am Soc Nephrol 2010;5:1064-71. [Crossref] [PubMed]
- Filipowicz R, Greene T, Wei G, et al. Associations of serum skeletal alkaline phosphatase with elevated C-reactive protein and mortality. Clin J Am Soc Nephrol 2013;8:26-32. [Crossref] [PubMed]
- Koehler EM, Sanna D, Hansen BE, et al. Serum liver enzymes are associated with all-cause mortality in an elderly population. Liver Int 2014;34:296-304. [Crossref] [PubMed]
- Fulks M, Stout RL, Dolan VF. Using liver enzymes as screening tests to predict mortality risk. J Insur Med 2008;40:191-203. [PubMed]
- Kunutsor SK, Bakker SJ, Kootstra-Ros JE, et al. Serum Alkaline Phosphatase and Risk of Incident Cardiovascular Disease: Interrelationship with High Sensitivity C-Reactive Protein. PLoS One 2015;10:e0132822 [Crossref] [PubMed]
- Bates CJ, Hamer M, Mishra GD. A study of relationships between bone-related vitamins and minerals, related risk markers, and subsequent mortality in older British people: the National Diet and Nutrition Survey of People Aged 65 Years and Over. Osteoporos Int 2012;23:457-66. [Crossref] [PubMed]
- Regidor DL, Kovesdy CP, Mehrotra R, et al. Serum alkaline phosphatase predicts mortality among maintenance hemodialysis patients. J Am Soc Nephrol 2008;19:2193-203. [Crossref] [PubMed]
- Haarhaus M, Brandenburg V, Kalantar-Zadeh K, et al. Alkaline phosphatase: a novel treatment target for cardiovascular disease in CKD. Nat Rev Nephrol 2017;13:429-42. [Crossref] [PubMed]
- Kunutsor SK, Apekey TA, Khan H. Liver enzymes and risk of cardiovascular disease in the general population: a meta-analysis of prospective cohort studies. Atherosclerosis 2014;236:7-17. [Crossref] [PubMed]
- Kunutsor SK, Apekey TA, Seddoh D, et al. Liver enzymes and risk of all-cause mortality in general populations: a systematic review and meta-analysis. Int J Epidemiol 2014;43:187-201. [Crossref] [PubMed]
- Li JW, Xu C, Fan Y, et al. Can serum levels of alkaline phosphatase and phosphate predict cardiovascular diseases and total mortality in individuals with preserved renal function? A systemic review and meta-analysis. PLoS One 2014;9:e102276 [Crossref] [PubMed]
- Shimizu Y, Imano H, Ohira T, et al. Alkaline phosphatase and risk of stroke among Japanese: the Circulatory Risk in Communities Study (CIRCS). J Stroke Cerebrovasc Dis 2013;22:1046-55. [Crossref] [PubMed]
- Bell S, Daskalopoulou M, Rapsomaniki E, et al. Association between clinically recorded alcohol consumption and initial presentation of 12 cardiovascular diseases: population based cohort study using linked health records. BMJ 2017;356:j909. [Crossref] [PubMed]
- Wieberdink R. Liver enzymes and the risk of intracerebral hemorrhage. Thesis: Determinants of cerebral infarction and intracranial hemorrhage -The Rotterdam Study 2012:121-32.
- Ryu WS, Lee SH, Kim CK, et al. Increased serum alkaline phosphatase as a predictor of long-term mortality after stroke. Neurology 2010;75:1995-2002. [Crossref] [PubMed]
- Kim J, Song TJ, Song D, et al. Serum alkaline phosphatase and phosphate in cerebral atherosclerosis and functional outcomes after cerebral infarction. Stroke 2013;44:3547-9. [Crossref] [PubMed]
- Lee HB, Kim J, Kim SH, et al. Association between Serum Alkaline Phosphatase Level and Cerebral Small Vessel Disease. PLoS One 2015;10:e0143355 [Crossref] [PubMed]
- Liu J, Wang D, Li J, et al. High Serum Alkaline Phosphatase Levels in Relation to Multi-Cerebral Microbleeds in Acute Ischemic Stroke Patients with Atrial Fibrillation and/or Rheumatic Heart Disease. Curr Neurovasc Res 2016;13:303-8. [Crossref] [PubMed]
- Liu J, Wang D, Li J, et al. Increased Serum Alkaline Phosphatase as a Predictor of Symptomatic Hemorrhagic Transformation in Ischemic Stroke Patients with Atrial Fibrillation and/or Rheumatic Heart Disease. J Stroke Cerebrovasc Dis 2016;25:2448-52. [Crossref] [PubMed]
- Ryu WS, Lee SH, Kim CK, et al. High serum alkaline phosphatase in relation to cerebral small vessel disease. Atherosclerosis 2014;232:313-8. [Crossref] [PubMed]
- Park JB, Kang DY, Yang HM, et al. Serum alkaline phosphatase is a predictor of mortality, myocardial infarction, or stent thrombosis after implantation of coronary drug-eluting stent. Eur Heart J 2013;34:920-31. [Crossref] [PubMed]
- Ndrepepa G, Xhepa E, Braun S, et al. Alkaline phosphatase and prognosis in patients with coronary artery disease. Eur J Clin Invest 2017;47:378-87. [Crossref] [PubMed]
- Ndrepepa G, Holdenrieder S, Xhepa E, et al. Prognostic value of alkaline phosphatase in patients with acute coronary syndromes. Clin Biochem 2017;50:828-34. [Crossref] [PubMed]
- Huseynov A, Baumann S, Becher T, et al. Liver and cholestatic parameters as prognostic biomarkers of in-hospital MACE in patients with STEMI. Eur J Clin Invest 2016;46:721-9. [Crossref] [PubMed]
- Nunes JP, Melao F, Godinho AR, et al. Plasma alkaline phosphatase and survival in diabetic patients with acute myocardial infarction. Ann Transl Med 2016;4:210. [Crossref] [PubMed]
- Oh PC, Lee K, Kim TH, et al. Prognostic impact of alkaline phosphatase measured at time of presentation in patients undergoing primary percutaneous coronary intervention for ST-segment elevation myocardial infarction. PLoS One 2017;12:e0171914 [Crossref] [PubMed]
- Ambrosy AP, Vaduganathan M, Huffman MD, et al. Clinical course and predictive value of liver function tests in patients hospitalized for worsening heart failure with reduced ejection fraction: an analysis of the EVEREST trial. Eur J Heart Fail 2012;14:302-11. [Crossref] [PubMed]
- Nikolaou M, Parissis J, Yilmaz MB, et al. Liver function abnormalities, clinical profile, and outcome in acute decompensated heart failure. Eur Heart J 2013;34:742-9. [Crossref] [PubMed]
- Allen LA, Felker GM, Pocock S, et al. Liver function abnormalities and outcome in patients with chronic heart failure: data from the Candesartan in Heart Failure: Assessment of Reduction in Mortality and Morbidity (CHARM) program. Eur J Heart Fail 2009;11:170-7. [Crossref] [PubMed]
- Samsky MD, Patel CB, DeWald TA, et al. Cardiohepatic interactions in heart failure: an overview and clinical implications. J Am Coll Cardiol 2013;61:2397-405. [Crossref] [PubMed]
- Mshe'el S, Bisharat N. Extremely high levels of serum alkaline phosphatase in patients with prolonged hepatic congestion. Eur J Gastroenterol Hepatol 2011;23:444. [Crossref] [PubMed]
- Shamban L, Patel B, Williams M. Significantly Elevated Liver Alkaline Phosphatase in Congestive Heart Failure. Gastroenterology Res 2014;7:64-8. [PubMed]
- Poelzl G, Ess M, Mussner-Seeber C, et al. Liver dysfunction in chronic heart failure: prevalence, characteristics and prognostic significance. Eur J Clin Invest 2012;42:153-63. [Crossref] [PubMed]
- Batin P, Wickens M, McEntegart D, et al. The importance of abnormalities of liver function tests in predicting mortality in chronic heart failure. Eur Heart J 1995;16:1613-8. [Crossref] [PubMed]
- Yamazoe M, Mizuno A, Nishi Y, et al. Serum alkaline phosphatase as a predictor of worsening renal function in patients with acute decompensated heart failure. J Cardiol 2016;67:412-7. [Crossref] [PubMed]
- Kerner A, Avizohar O, Sella R, et al. Association between elevated liver enzymes and C-reactive protein: possible hepatic contribution to systemic inflammation in the metabolic syndrome. Arterioscler Thromb Vasc Biol 2005;25:193-7. [PubMed]
- Cheung BM, Ong KL, Cheung RV, et al. Association between plasma alkaline phosphatase and C-reactive protein in Hong Kong Chinese. Clin Chem Lab Med 2008;46:523-7. [Crossref] [PubMed]
- Webber M, Krishnan A, Thomas NG, et al. Association between serum alkaline phosphatase and C-reactive protein in the United States National Health and Nutrition Examination Survey 2005-2006. Clin Chem Lab Med 2010;48:167-73. [Crossref] [PubMed]
- Krishnamurthy VR, Baird BC, Wei G, et al. Associations of serum alkaline phosphatase with metabolic syndrome and mortality. Am J Med 2011;124:566.e1-7. [Crossref] [PubMed]
- Zhang L, Ma X, Jiang Z, et al. Liver enzymes and metabolic syndrome: a large-scale case-control study. Oncotarget 2015;6:26782-8. [Crossref] [PubMed]
- Hansson GK. Inflammation, atherosclerosis, and coronary artery disease. N Engl J Med 2005;352:1685-95. [Crossref] [PubMed]
- Jean G, Charra B, Chazot C. Vitamin D deficiency and associated factors in hemodialysis patients. J Ren Nutr 2008;18:395-9. [Crossref] [PubMed]
- Giovannucci E, Liu Y, Hollis BW, et al. 25-hydroxyvitamin D and risk of myocardial infarction in men: a prospective study. Arch Intern Med 2008;168:1174-80. [Crossref] [PubMed]
- Aleksova A, Belfiore R, Carriere C, et al. Vitamin D Deficiency in Patients with Acute Myocardial Infarction: An Italian Single-Center Study. Int J Vitam Nutr Res 2015;85:23-30. [Crossref] [PubMed]
- De Metrio M, Milazzo V, Rubino M, et al. Vitamin D plasma levels and in-hospital and 1-year outcomes in acute coronary syndromes: a prospective study. Medicine (Baltimore) 2015;94:e857 [Crossref] [PubMed]
- Zittermann A, Schleithoff SS, Koerfer R. Putting cardiovascular disease and vitamin D insufficiency into perspective. Br J Nutr 2005;94:483-92. [Crossref] [PubMed]
- Brewer LC, Michos ED, Reis JP. Vitamin D in atherosclerosis, vascular disease, and endothelial function. Curr Drug Targets 2011;12:54-60. [Crossref] [PubMed]
- Milazzo V, De Metrio M, Cosentino N, et al. Vitamin D and acute myocardial infarction. World J Cardiol 2017;9:14-20. [Crossref] [PubMed]
- Schwetz V, Trummer C, Pandis M, et al. Effects of Vitamin D Supplementation on Bone Turnover Markers: A Randomized Controlled Trial. Nutrients 2017;9. [PubMed]
- Targher G, Bertolini L, Padovani R, et al. Prevalence of nonalcoholic fatty liver disease and its association with cardiovascular disease among type 2 diabetic patients. Diabetes Care 2007;30:1212-8. [Crossref] [PubMed]
- Fargion S, Porzio M, Fracanzani AL. Nonalcoholic fatty liver disease and vascular disease: state-of-the-art. World J Gastroenterol 2014;20:13306-24. [Crossref] [PubMed]
- Stepanova M, Younossi ZM. Independent association between nonalcoholic fatty liver disease and cardiovascular disease in the US population. Clin Gastroenterol Hepatol 2012;10:646-50. [Crossref] [PubMed]
- Kunutsor SK, Bakker SJL, Blokzijl H, et al. Associations of the fatty liver and hepatic steatosis indices with risk of cardiovascular disease: Interrelationship with age. Clin Chim Acta 2017;466:54-60. [Crossref] [PubMed]
- Schoppet M, Shanahan CM. Role for alkaline phosphatase as an inducer of vascular calcification in renal failure? Kidney Int 2008;73:989-91. [Crossref] [PubMed]
- Zhu D, Mackenzie NC, Farquharson C, et al. Mechanisms and clinical consequences of vascular calcification. Front Endocrinol (Lausanne) 2012;3:95. [Crossref] [PubMed]
- Johnson RC, Leopold JA, Loscalzo J. Vascular calcification: pathobiological mechanisms and clinical implications. Circ Res 2006;99:1044-59. [Crossref] [PubMed]
- Fakhry M, Roszkowska M, Briolay A, et al. TNAP stimulates vascular smooth muscle cell trans-differentiation into chondrocytes through calcium deposition and BMP-2 activation: Possible implication in atherosclerotic plaque stability. Biochim Biophys Acta 2017;1863:643-53.
- Shanahan CM, Cary NR, Salisbury JR, et al. Medial localization of mineralization-regulating proteins in association with Monckeberg's sclerosis: evidence for smooth muscle cell-mediated vascular calcification. Circulation 1999;100:2168-76. [Crossref] [PubMed]
- Tang FT, Chen SR, Wu XQ, et al. Hypercholesterolemia accelerates vascular calcification induced by excessive vitamin D via oxidative stress. Calcif Tissue Int 2006;79:326-39. [Crossref] [PubMed]
- Lomashvili KA, Garg P, Narisawa S, et al. Upregulation of alkaline phosphatase and pyrophosphate hydrolysis: potential mechanism for uremic vascular calcification. Kidney Int 2008;73:1024-30. [Crossref] [PubMed]
- Narisawa S, Harmey D, Yadav MC, et al. Novel inhibitors of alkaline phosphatase suppress vascular smooth muscle cell calcification. J Bone Miner Res 2007;22:1700-10. [Crossref] [PubMed]
- Sheen CR, Kuss P, Narisawa S, et al. Pathophysiological role of vascular smooth muscle alkaline phosphatase in medial artery calcification. J Bone Miner Res 2015;30:824-36. [Crossref] [PubMed]
- Dao HH, Essalihi R, Bouvet C, et al. Evolution and modulation of age-related medial elastocalcinosis: impact on large artery stiffness and isolated systolic hypertension. Cardiovasc Res 2005;66:307-17. [Crossref] [PubMed]
- Speer MY, Giachelli CM. Regulation of cardiovascular calcification. Cardiovasc Pathol 2004;13:63-70. [Crossref] [PubMed]
- London GM. Arteriosclerosis and arterial calcifications in chronic kidney insufficiency. Nephrol Ther 2005;1:S351-4. [PubMed]
- Niskanen L, Siitonen O, Suhonen M, et al. Medial artery calcification predicts cardiovascular mortality in patients with NIDDM. Diabetes Care 1994;17:1252-6. [Crossref] [PubMed]
- Hughes-Austin JM, Dominguez A 3rd, Allison MA, et al. Relationship of Coronary Calcium on Standard Chest CT Scans With Mortality. JACC Cardiovasc Imaging 2016;9:152-9. [Crossref] [PubMed]
- Virmani R, Burke AP, Farb A. Plaque morphology in sudden coronary death. Cardiologia 1998;43:267-71. [PubMed]
- Abedin M, Tintut Y, Demer LL. Vascular calcification: mechanisms and clinical ramifications. Arterioscler Thromb Vasc Biol 2004;24:1161-70. [Crossref] [PubMed]
- Hutcheson JD, Maldonado N, Aikawa E. Small entities with large impact: microcalcifications and atherosclerotic plaque vulnerability. Curr Opin Lipidol 2014;25:327-32. [Crossref] [PubMed]
- Sahin I, Karabulut A, Gungor B, et al. Correlation between the serum alkaline phosphatase level and the severity of coronary artery disease. Coron Artery Dis 2014;25:349-52. [Crossref] [PubMed]
- Karabulut A, Sahin I, Avci II, et al. Impact of serum alkaline phosphatase level on coronary collateral circulation. Kardiol Pol 2014;72:1388-93. [Crossref] [PubMed]
- Karabulut A, Sahin I, Ilker Avci I, et al. Impact of serum alkaline phosphatase level on the pathophysiologic mechanism of contrast-induced nephropathy. Kardiol Pol 2014;72:977-82. [Crossref] [PubMed]
- Cseprekal O, Kis E, Degi AA, et al. Bone metabolism and arterial stiffness after renal transplantation. Kidney Blood Press Res 2014;39:507-15. [Crossref] [PubMed]
- Savinov AY, Salehi M, Yadav MC, et al. Transgenic Overexpression of Tissue-Nonspecific Alkaline Phosphatase (TNAP) in Vascular Endothelium Results in Generalized Arterial Calcification. J Am Heart Assoc 2015;4:e002499 [Crossref] [PubMed]
- Perticone F, Perticone M, Maio R, et al. Serum alkaline phosphatase negatively affects endothelium-dependent vasodilation in naive hypertensive patients. Hypertension 2015;66:874-80. [Crossref] [PubMed]
- Ali AT, Penny CB, Paiker JE, et al. Alkaline phosphatase is involved in the control of adipogenesis in the murine preadipocyte cell line, 3T3-L1. Clin Chim Acta 2005;354:101-9. [Crossref] [PubMed]
- Hernandez-Mosqueira C, Velez-delValle C, Kuri-Harcuch W. Tissue alkaline phosphatase is involved in lipid metabolism and gene expression and secretion of adipokines in adipocytes. Biochim Biophys Acta 2015;1850:2485-96.
- Grethen E, Hill KM, Jones R, et al. Serum leptin, parathyroid hormone, 1,25-dihydroxyvitamin D, fibroblast growth factor 23, bone alkaline phosphatase, and sclerostin relationships in obesity. J Clin Endocrinol Metab 2012;97:1655-62. [Crossref] [PubMed]
- Gutierrez OM, Mannstadt M, Isakova T, et al. Fibroblast growth factor 23 and mortality among patients undergoing hemodialysis. N Engl J Med 2008;359:584-92. [Crossref] [PubMed]
- Dey T, Gogoi K, Unni B, et al. Role of environmental pollutants in liver physiology: special references to peoples living in the oil drilling sites of Assam. PLoS One 2015;10:e0123370 [Crossref] [PubMed]
Cite this article as: Ndrepepa G. Alkaline phosphatase and cardiovascular disease. J Lab Precis Med 2017;2:83.