Circulating biomarkers in heart failure: diagnostic and prognostic importance
Introduction
Heart failure (HF) is a major cause of premature death due to cardiovascular (CV) reasons in general population and in individuals with recently established CV disease (1). The prevalence of acute and chronic HF is steadily increasing worldwide, while there is expressive advance in our understanding of basic mechanisms of nature evolution of the disease and principles of prevention and treatment at different stages of the cardiac dysfunction shaping. However, for two past decades in most of developing and developed countries there is growth of economic burden of patients’ families and medical care systems and as well as an increase of primary and urgent admission rate of the patients with suspected acute HF and decompensated chronic HF (2). Nevertheless, there are significant differences between sexes, races, and individuals at different ages and numerous comorbidities in prevalence, etiology, and epidemiology, presentation of clinical findings and phenotypes of HF, prognosis, and response to the disease treatment (3). Although contemporary clinical guidelines that were recently published clearly described the main principles of diagnosis, prevention and treatment of acute and chronic HF, a personified risk stratification of the disease remained to be under discussion (4). As a fact, numerous biological markers that are molecular indicators of several pathophysiological stages of nature evolution HF could become a powerful and reliable tool for more exact risk prognostication and accurate predictors of treatment response (5). The aim of the chapter is to summarize knowledge regarding the promising role of biological biomarker in diagnosis, risk stratification and personifying treatment strategy in patients with acute and chronic HF.
Conventionally used biomarkers of heart failure
A biomarker is a qualitative and quantitative biological substance/characteristic/parameter(s) that defines a certain pathological condition and may give indications on disease activity or severity and the type of therapy that should be administered to the patient. In 1998, the National Institutes of Health Biomarkers Definitions Working Group defined biomarker as “a characteristic that is objectively measured and evaluated as an indicator of normal biological processes, pathogenic processes, or pharmacologic responses to a therapeutic intervention” (6). The International Program on Chemical Safety, led by the World Health Organization (WHO) and in coordination with the United Nations and the International Labor Organization, has defined a biomarker as “any substance, structure, or process that can be measured in the body, or its products and influences or predicts the incidence of outcome or disease” (7). The National Academy of Sciences defines a biomarker as an indicator that signals events in biological samples or systems. Finally, experts of FDA (Food and Drug Association, USA) biomarkers can be identified as a broad subcategory of medical signs, which, by accurate measures and reproducibility, can provide an objective suggestion of the medical state examined from outside the patient (7). All these terms, definitions, and characteristics were proposed to describe a biomarker, indicating that it may have the greatest value in early efficacy and safety evaluations, such as in vitro studies in tissue samples, in vivo studies in animal models, and early-phase clinical trials. Indeed, there are numerous of biomarkers, which reflect several pathophysiological stages of HF and allow stratifying individuals at risk (Figure 1).
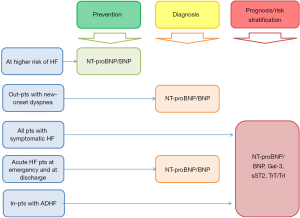
There has been increasing interest in diagnostic and management strategies of HF based on biomarkers in US and in countries in Europe. Moreover, the real market of these biological markers appears to be extremal growth for last decade. On the one hand, the implementation of biomarkers use in the evidence-based clinical practices can improve efficiency and effectiveness of public health management. On the other hand, all these steps undoubtedly associated with increased cost of new diagnostic and treatment approaches based on biomarker(s) measure. To be able to shape personalized medical care to HF patients, it is extremely important to know which biomarkers are better corresponded to the treatment goals including clinical symptoms, functional status, quality of life, survival and admission to the hospital (8). Contemporary clinical guidelines described what kind of biological markers are necessary to predict most of these goals. The family of natriuretic peptides (NPs) are recommended biomarkers for routine clinical practice to risk stratification and diagnosis of the HF. In contrast, galectin-3, soluble suppressor of tumorigenicity-2 receptor (sST2) and high-sensitivity cardiac troponins can be discussed as promising candidates for improving prediction and risk stratification in HF patients (Table 1). Interestingly, recent ESC HF clinical guidelines introduced a new HF phenotype based on measure of left ventricular (LV) ejection fraction (EF) and determined the HF with mid-range EF (HFmrEF). Novel HF phenotype falls between the HF with reduced LVEF (HFrEF) and HF with preserved LVEF (HFpEF) (Table 2). The biomarkers’ characteristics of the HFmrEF are became uncertain and require to be investigated in details, although there is suggestion that biomarkers commonly used for risk prediction of HFrEF could be more valuable for HFmrEF prognostication. Commonly available biomarkers primarily help to establish or refute the diagnosis of HF, help to determine the HF severity, and identify adverse consequences of treatment. Overall, in any clinical scenario biological markers as a two/tree steps’ algorithm could improve a conventional risk stratification based on clinical criteria and some instrumental parameters in dyspneic individuals with mild diastolic dysfunction (Table 3). In fact, the implementation of echocardiographic evaluation of signs/symptoms, resting LVEF and E/e', abnormal diastolic response to exercise followed by the assessment of NPs, galectin-3 and sST2 may improve the diagnosis and prognostic assessment of asymptomatic individuals with HFpEF/HFmrEF and patients with suspected HFpEF who are unable to perform a diagnostic exercise test (9,11). In this context, all these biomarkers deserve to be routinely assessed in subsequent validation studies. However, most evidence of biomarkers as of diagnostic tool with discriminative value has applied for acute and chronic HFrEF individuals as well as chronic HFpEF. In fact, personalized risk prediction based on biomarker measure in acute HF patients remains to be challenged.
Table 1
Suggestions for use | Patients | COR | LOE | References |
---|---|---|---|---|
NPs | ||||
Rule-in or support of initial working diagnosis | Patients with suspected HF in non-acute setting condition with dyspnea | I | A | (4,9) |
Patients with suspected HF, when the etiology of dyspnea is unclear | I | A | (5,10) | |
Patients with suspected HF in acute setting condition | IIb | C | (11) | |
Exclusion of important cardiac dysfunction | Outpatients with uncertain signs and symptoms of HF | I | A | (10) |
Prognosis of HF | Outpatients / inpatients with established HF | I | A | (10) |
Patients who were admitted to the hospital with acute HF | I | A | (10) | |
Post-discharged HF patients | IIa | B | (12) | |
Prevent development of LV dysfunction or new-onset HF | Patients at risk of HF | IIa | B | (10,13) |
Target therapy | Outpatients with established HF in euvolemic condition | IIa | B | (10,12,14) |
Biomarkers of myocardial injury (cardiac troponins) | ||||
Risk stratification | Patients with established HF | I | A | (10,15,16) |
Patients who were admitted to the hospital with acute HF | I | A | (10,17) | |
Biomarkers of myocardial fibrosis (galectin-3) | ||||
Risk stratification | Outpatients with established chronic HF | IIb | B | (18,19) |
Inpatients with established acute and chronic HF | IIb | A | (20-22) | |
Post-discharged patients | IIa | B | (23) | |
sST2 | ||||
Prognosis of HF | Outpatients / inpatients with established HF | I | A | (23) |
Patients who were admitted to the hospital with acute HF | I | A | (19,24,25) | |
Post-discharged patients | IIa | B | (25) |
HF, heart failure; NPs, natriuretic peptides; BNP, brain NP; NT-proBNP, N-terminal fragment of brain NP; sST2, soluble suppressor of tumorigenicity-2; MR-proANP, mid-regional pro-atrial NP; COR, classes of recommendations; LOE, level of evidence.
Table 2
Characteristics of HF | Phenotypes of chronic HF | ||
---|---|---|---|
HFrEF | HFpEF | HFmrEF | |
Symptoms and signs of HF | Symptoms and signs relate to hypervolemic state, fluid retention, diuretics’ use | ||
LVEF | < 40% | >50% | 40–49% |
NP level(s) | Extremely elevated | Mild-to-moderate elevated | Mild-to-moderate elevated |
Relevant structure heart disease | CAD, myocardial infarction, dilated cardiomyopathy, myocarditis, tachycardiomyopathy | LVH, hypertrophic and restrictive cardiomyopathies, CAD, infiltrative diseases, | LVH, CAD, pulmonary hypertension, diabetes-induced cardiomyopathy, tachycardiomyopathy |
Relevant systolic dysfunction/diastolic filling abnormalities | Primary severe systolic dysfunction with diastolic filling abnormalities | Primary diastolic heart failure* | Primary mild systolic dysfunction with diastolic filling abnormalities |
Key structural alterations of LA | LAVI >34 mL/m2 | LAVI >34 mL/m2 | LAVI >34 mL/m2 |
Notes: NP(s) levels mean BNP >35 pg/mL and/or NT-proBNP >125 pg/mL; *, diastolic dysfunction is determined if four parameters of annular e' velocity are presented their abnormal cutoff values are: septal e' <7 cm/sec, lateral e' <10 cm/sec, average E/e' ratio >14, LA volume index >34 mL/m2, and peak TR velocity >2.8 m/sec. On the basis of the writing group’s collective expert opinion, average E/e' ratio is recommended for simplification. HFrEF, heart failure with reduced left ventricular ejection fraction; HFmrEF, heart failure with mid-range left ventricular ejection fraction; HFpEF, heart failure with preserved left ventricular ejection fraction; CAD, coronary artery disease; LVH, left ventricular hypertrophy; LVEF, left ventricular ejection fraction; LA, left atrial; TR, tricuspidal rout; LAVI, left atrial volume index.
Table 3
Predictors | Phenotype of HF | Biomarkers | Diagnostic improvement | Predictive improvement |
---|---|---|---|---|
Clinical signs and symptoms (edema, dyspnea, fatigue, palpitation, low exercise tolerance, fluid retention) | Any | Elevated BNP >35 pg/mL and/or NT-proBNP >125 pg/mL | Increase the likelihood of the diagnosis | Prediction of the risk of admission |
Elevated BNP <35 pg/mL and/or NT-proBNP <125 pg/mL | Ruling-out of the diagnosis | – | ||
Suspected HFrEF | Elevated galectin-3 | Ruling-in of the diagnosis | Prediction of the risk of death | |
HFrEF, HFmrEF, HFpEF (?) | Elevated sST2 | Ruling-in of the diagnosis | Prediction of the risk of death and admission | |
Resting LVEF >40% | HFpEF, HFmrEF | BNP >35 pg/mL and/or NT-proBNP >125 pg/mL | Confirm diagnosis | Prediction of cardiovascular death or admission |
Acute HF/ADHF | Elevated tropinin T above 99 percentile of reference range | – | ||
Resting E/e' <14 | HFpEF | Elevated BNP >35 pg/mL and/or NT-proBNP >125 pg/mL | Rule-in of diagnosis | Prediction of cardiovascular death or admission |
Abnormal diastolic response to exercise (increase in left ventricular filling pressure) | Asymptomatic mild diastolic dysfunction | Elevated BNP >35 pg/mL and/or NT-proBNP >125 pg/mL | Rule-in of diagnosis | Prediction of cardiovascular admission and death |
HFpEF | Elevated galectin-3 >1.17 ng/mL | Rule-in of diagnosis | Prediction of composite outcome of cardiovascular admission or death |
? means lack of data. ADHF, acutely decompensated heart failure; LVEF, left ventricular ejection fraction; BNP, brain natriuretic peptide; HFpEF, heart failure with preserved ejection fraction; E/e', resting peak early diastolic mitral inflow velocity/peak early diastolic mitral annular velocity ratio.
NPs
NPs predominantly brain NPs (BNP) and NT-proBNP were recommended by the European Society of Cardiology and American Heart Association for exclusion HF in patients with acute dyspnea, and now they are incorporated into contemporary clinical guidelines as powerful tool for diagnosis, prognosis, risk stratification, and even NPs-guided therapy (1,6). Atrial NP (ANP) and BNP are recognized key regulators of systemic blood pressure, water and salt homeostasis and they are biomarkers of mechanical distress and cardiac wall stretching including acute and chronic fluid overload. Additionally, ANP release from atrial granules upon acute volume overload versus increased synthesis by cardiac myocytes in chronic fluid overload, whereas BNP does not accumulate before any stimuli. In contrast to ANP and BNP, C-type of NP (CNP) is released from endothelial cells and renal cells in response to mechanical stimuli, activation by shear stress and inflammatory cytokines (8).
Both ANP and BNP may counteract renin-angiotensin-aldosterone system, systemic sympathetic activity and other neurohormonal factors including endothelin and vasopressin. ANP and BNP binds with appropriate NP receptor type A, which are expressed at the surfaces of the target cells and cooperated with cGMP. The main biological effects of NPs are diuresis, natriuresis, increasing glomerular filtration rate, decrease of circulating plasma volume and blood pressure regulation. Moreover, NPs may ensure indirect anti-proliferative activity and mild anti-mutagenic effect that are able to support reversion of hypertrophy of cardiac and vascular walls (7). Therefore, they are produced by cardiac myocytes due to ischemia, necrosis, inflammation, metabolic and toxic damage, and membrane instability (7,8).
Elevated circulating level of NPs particularly BNP and NT-proBNP correlated well with the HF severity and are practically useful biomarkers for the HF diagnosis as well as prognostic markers for CV risk including death due to all causes and HF-related events (14,26). Indeed, in patients with established HFrEF the levels of BNP and NT-proBNP are typically >100 and >250 pg/mL, respectively (12). However, after implementation into routine clinical practice novel drugs—angiotensin receptor blockers/neprilysin inhibitors (ARNI)—this is necessarily indicating the new criterion for NPs’ levels with prediction value (10,12). In fact, although NP-based guided therapy of HF has been intensively investigated, but the clinical advantages of the approach requires being studied (13).
Galectin-3
Galectin-3 is a soluble form of the β-galactoside-binding protein that is released from activated mononuclears and other antigen presenting cells due to antigen stimulation. The main biological role of galectin-3 is to activate the fibroblasts and support extravascular accumulation of collagen that lead to fibrosis in target organs including heart and kidney (27). Although the myocardial fibrosis and cardiac injury could be source of cardiac failure, but markers typical of these events are not per se markers of HF. However, galectin-3 appears to be a “cumulative” biomarkers that reflect per se interrelation between inflammation and tissue remodeling including fibrosis. Indeed, galectin-3 has been served as a prognostic clinical biomarker in HF and other diseases associated with cardiac remodeling and nephropathy, such as atherosclerosis, stroke, diabetes mellitus, vasculitis, and connective tissue diseases (20,21,28). Although galectin-3 was found in elevated concentrations in a serum of the patients with acute and chronic HF regardless of LVEF, there was positive association between galectin-3 level and NT-proBNP level, the estimated glomerular filtration rate but not with age and serum cardiac troponins in individuals with HFrEF (18). In fact, peak concentrations of galectin-3 were related to activity of inflammation, worsening tissue repair and intensity of fibrogenesis in patients with ischemic and non-ischemic chronic HF, but serial repeated measure of the levels of galectin-3 did not exhibit advantages before single measure of this marker in cohort studies (22,23). In contrast, the TRIUMPH (Translational Initiative on Unique and Novel Strategies for Management of Patients with Heart Failure) study serial measurements of galectin-3 levels were a strong independent predictor of clinical outcomes in acute HF patients (24). Interestingly, galectin-3 was not superior to NT-proBNP, sST2, growth differentiation factor (GDF)-15 or high-sensitive C-reactive protein (hsCRP) in prediction of CV mortality and HF-elated clinical outcomes including death, while the combination of both galectin-3 and NT-proBNP was more accurate in predicting HF-related death compared to either of other biomarkers alone (23). Whether galectin-3 is not yet predictive biomarker, but biological target for prevention of HF and extracellular fibrotic remodeling, is not fully understood.
Soluble suppressor of tumorigenicity-2 receptor
Soluble suppressor of tumorigenicity-2 receptor (sST2) belongs to the interleukin (IL)-1 receptor family members. The ST2 consists in two comprising isoforms named membrane-bound (ST2L) and soluble (sST2) isoforms. sST2 binds with its ligand recognized as IL-33 and support production of Th1-related cytokines (such as tumor necrosis factor-alpha) that may play a pivotal role in inflammation, cardiac hypertrophy and remodeling, fibrotic accumulation and necrosis (8). However, there is evidence that sST2 is not just fibrotic and inflammatory biomarkers, but it might be a predictor of the clinical outcomes and nature evolution of HF.
Elevated serum levels of sST2 were found in patients with acute and chronic HF regardless of LVEF. Additionally, levels of sST2 in chronic HF individuals demonstrated close positive association with NYHA HF functional class and both levels of BNP, hs-CRP and GDF15 (19,25). Peak level of sST2 was served as powerful predictor of all-cause mortality, CV death and clinical outcomes in HFrEF/HFpEF and probably in HFmrEF patients (15,17,19). Whether serial measures of sST2 levels are independently predict cardiac fibrosis, vascular remodelling and the progression of HF is not clear.
Biomarkers of myocardial injury
Development and progression of HF strongly relates to direct and indirect damages of cardiac cells by effect of etiology factors of cardiac dysfunction (i.e., ischemia/necrosis, inflammation, hypoxia, hypertrophy, fibrosis) as well as by other factors contributing in pathogenesis of HF (i.e., biomechanical stress due to cardiac remodeling, iron deficiency, oxidative stress/mitochondrial dysfunction). Biomarkers of myocardial injury may be detected in peripheral blood in exaggerated concentration as result in leakage through cardiac cell membranes and due to injury of cells. However, regardless the main cause of cell dysfunction, biomarkers of cardiac cell injury reflect a wide range of pathophysiological process: from instability of lipid layers of membrane due to lipid peroxidation to destroying cell due to necrosis/apoptosis (8).
The wide range of myocardial injury biomarkers, such as cardiac troponins T and I, myoglobin, heart type of fatty acid binding protein, glutathione transferase P1, appeared to be promising predictors of HF-related clinical outcomes and CV death (16). However, high-sensitive cardiac troponins are recommended to improve stratification of the HF patients (29,30).
Interpretative limitations in use of traditional HF biomarkers
There is a large body of evidence regarding the controversial role of NPs in personalized treatment of HF. Indeed, the serum levels of NPs related to age of the patients, co-morbidities, kidney and metabolic clearance (31-35). It is well known that NPs are undergone modifications due to neprilysation, glycosylation, methylation, and oxidation depending on individual particularities (age, kidney clearance, liver function, drug abuse etc.) (32-34) that leads to higher individual biological variability of serum levels of NPs (35-37). Therefore, there is a list of the diseases associated with increased level of NPs beyond HF development (Table 4).
Table 4
Diseases | Types of changes | Primary causes for NP evolution |
---|---|---|
Acute and chronic HF | ↑↑↑ | Over-production due to myocardial wall stretching / fluid overload |
MI/ACS | ↑↑ | Cardiac injury |
Atrial fibrillation/atrial flutter | ↑↑ | Leakage through cardiac myocyte membrane |
Myocardities/cardiomyopathy | ↑–↑↑↑ | Cardiac injury |
Cardiac hypertrophy | ↑ | Leakage through cardiac myocyte membrane |
Cardioversion | ↑ | Cardiac injury |
Cancer chemotherapy | ↑ | Toxic-metabolic myocardial insults |
Valvular and pericardial disease | ↑–↑↑ | Leakage through cardiac myocyte membrane |
Pulmonary hypertension | ↑–↑↑ | Leakage through cardiac myocyte membrane |
Cardiac surgery | ↑ | Leakage through cardiac myocyte membrane |
Aging | ↑ | Lowered kidney clearance |
DM | ↑–↑↑ | Lowered kidney clearance |
COPD | ↑↑ | Myocardial wall stretching |
Obesity | ↓ | Increased degradation by enzymes (glycosylation for NT-poBNP, neprilysin for BNP) |
Anemia | ↑ | Leakage through cardiac myocyte membrane |
Renal failure | ↑ | Lowered kidney clearance |
Critical illness, bacterial sepsis, severe burns | ↑–↑↑ | Lowered kidney clearance |
↑, mild increase; ↑↑, moderate increase; ↑↑↑, severe increase; ↓, decrease. NP, natriuretic peptide; HF, heart failure; ACS, acute coronary syndrome; MI, myocardial infarction; COPD, chronic obstructive pulmonary disease; DM, diabetes mellitus.
The main cause of individual variability of serum biomarker concentration is glomerular filtration rate (GFR). Several biomarkers, such as NPs, galectin-3, GDF-15, sST2 and cardiac troponins being independent predictor of all-cause mortality, CV death and HF death, demonstrated close inverse relation to decreased GFR and increased aged (38-40). Consequently, lowered GFR and older age should be paid into account on interpretation of biomarker levels (41). At the same time, galectin-3 was found as biomarker with the lowest individual biological variability (42-44), whereas sST2 was not associated with age, sex and cardiac hemodynamic characteristics (45-48). Thus, there is no biomarker without several limitations for interpretation in practical manner, but multiple biomarker models might have higher accuracy in HF outcomes prediction and lover relation to age, sex, co-morbidities and GFR (48,49).
Novel biomarkers for HF management
The exploration of brand new biomarkers and investigation of novel multiple biomarker models appears to be promising methods to improve diagnostic and predictive value of currently used score of HF stratification (50,51). Table 5 is reported some promising biomarkers reflected several faces of pathogenesis of HF that could be useful in HF stratification in the future.
Table 5
Related pathophysiological processes in HF | HF phenotype | Biomarkers | Relevance to clinical outcomes in HF | ||
---|---|---|---|---|---|
Mortality | Hospitalization | Risk of HF deterioration | |||
Myocardial biochemical stress | Any | MR-proANP | + | + | + |
Neurohumoral activation | HFrEF | Copeptin | + | + | + |
HFrEF | CT-proET-1 | − | − | + | |
HFrEF | ADM / MR-proADM | + | + | + | |
Myocardial fibrosis | HFpEF/HFmrEF | PICP | + | + | + |
HFpEF/HFmrEF | CITP | + | + | + | |
HFpEF/HFmrEF | PIIINP | + | + | + | |
HFpEF, HFmrEF | MMPs | + | + | − | |
Myocardial necrosis | Any | hFABP | + | + | − |
Any | GSTP1 | + | + | − | |
Vascular remodeling | Any | OPN | + | + | − |
HFpEF/HFmrEF | OPG | + | + | − | |
Any | Signature of miRNAs | + | + | + | |
Inflammation | HFrEF | hs-CRP | + | + | + |
HFrEF | Procalcitonin | + | + | + | |
HFrEF | GDF-15 | + | + | + | |
Oxidative stress | HFrEF | Uric acid | + | + | + |
HFrEF | Myeloperoxidase | + | + | + | |
HFpEF/HFmrEF | Ceruloplasmin | + | + | + | |
HFpEF/HFmrEF | 8-OHdG | + | + | + | |
HFpEF/HFmrEF | Trx1 | + | + | + | |
Renal dysfunction | HFrEF | Cystatin C | + | + | + |
HFrEF | NGAL | + | + | + | |
Metabolomic state | HFrEF | Signature of metabolomics (fatty and amine acids, Krebs cycle components, DNAs, lipids, glucose, variable very-long chain carbons, proteins, hormones, enzymes etc.) | + | + | + |
Endothelial dysfunction | HFpEF, HFrEF, HFmrEF (?) | Endothelial precursors | + | + | + |
Any | EMVs | + | + | + |
+, an effect has now confirmed; −, an effect has not now confirmed; ?, the relation between HF phenotypes and endothelial precursors are not strong investigated. ADHF, acutely decompensated heart failure; MR-proANP, mid-regional pro atrial natriuretic peptide; ADM, adrenomedullin; MR-proADM, mid-regional pro-adrenomedullin; PICP, carboxy terminal propeptide; CT-proET-1, C-terminal-pro-endothelin-1; CITP, carboxy-terminal telopeptide; PIIINP, amino-terminal peptide of procollagen type III; HF, heart failure; hs-CRP, high-sensitive C-reactive protein; hFABP, high-sensitive fatty acid binding protein; GDF, growth differentiation factor; EMPs, endothelial micro vesicles; MMP, matrix metalloproteinase; NGAL, neutrophil gelatinase-associated lipocalin; 8-OHdG, 8-hydroxy-2'-deoxyguanosine; Trx1, thioredoxin 1; GSTP1; glutathione transferase P1
Procalcitonin
Procalcitonin is determined a propeptide of calcitonin, which is normally produced by the parafollicular C cells of the thyroid gland (52). Procalcitonin/calcitonin axis is essential for regulation of calcium homeostasis and immunity (53). Recent preclinical and clinical studies have shown that extra-thyroidal production of procalcitonin markedly increases in cases of systemic inflammatory reaction, severe infections (viral, bacterial, fungal and parasitic), and shock (54,55). Although serial measurements of procalcitonin are recommended to discriminate of in-hospital mortality in various diseases associated with pro-inflammatory activation (pneumonia, chronic obstructive pulmonary disease, acute respiratory tract infections, sepsis, etc.), lack of strong evidence that the serum procalcitonin levels could be reliable indicator for chronic HF with predictive value (55). The large clinical trials are required to clearly explain the suggestion and fetch more evidence regarding a predictive role of procalcitonin in exacerbated HF individuals.
Copeptin
Copeptin is C-terminal derivate of the arginine vasopressin that normally acts as regulator of water and electrolyte homeostasis (56). Although plasma levels of copeptin are very variable and tightly relate to blood/urine osmolality, copeptin appears to be in higher concentrations in sever hypertension, stroke, acute and chronic HF, myocardial infarction, diabetes mellitus, advanced kidney diseases, and in critical conditions. As quantitative biomarker of endogenous biomechanical stress elevated level of copeptin was found in close positive association with increased CV mortality and CV disease in out-patients and all-cause mortality in critical ill patients (57). There is a large body of evidence regarding that the serial measurements of copeptin level may be provide an important information for discrimination of a risk of all-cause mortality, HF-related outcomes and CV events and diseases (58-61). Although both increased NT-proBNP levels and copeptin levels were recognized significant independent predictors of adverse clinical outcomes in HF, the role of dual markers’ contribution in HF risk stratification remains to be challenged (62-65).
The heart type of fatty acid binding protein
The heart type of fatty acid binding protein (hFABP) is normally essential for the long-chain fatty acids re-uptake, regulation of calcium homeostasis in cardiomyocytes and mediating inflammatory reaction (66). Because hFABP is tissue-specific biomarker of myocardial injury and necrosis, it is reserved as predictor of myocardial infarction at the early hours of development of the disease. Recent studies have shown that circulating levels of hFABP are elevated in cardiac dysfunction and closely predicted CV outcomes and HF-related events in in-patients especially in those who had fluid retention and lung congestion (66-68). Although elevated serum level of hFABP exhibited better prognostic information on survival in individuals with acute and advanced HF when compared to NPs, cardiac troponins and even galectin-3 taken alone, there is confusing in improved precision of entire predictive model after incorporating hFABP to NPs and/or galectin-3 (66,68).
GDF-15
GDF-15 is multifunctional cytokine that belongs to the transforming growth factor-β superfamily (69). GDF-15 is normally expressed in various cells including immune cells, fibroblasts, myocardial cells, endothelial cells, and mononuclears. Additionally, GDF-15 is actively secreted into circulation by cardiac myocytes due to stretching and biochemical stress (61,69,70).
Serum levels of GDF-15 associated with increased risk of all-cause death independently to age, clinical signs and symptoms of cardiac dysfunction, LFEF, renal function and NPs in HF (71). Interestingly, in individuals with acute HF the serum levels of GDF-15 were not better to NPs and galectin-3 taken alone in accuracy to predict clinical outcomes including HF-related death and re-admission due to HF decompensation shortly after previous discharge (72). In contrast, GDF-15 could be superior to sST2 in prediction of fatal arrhythmic events and all-cause mortality in non-ischemic CH (9,11). The out-patients with chronic HFrHF/HFpEF/HFmrEF may be candidates to multiple predictive biomarker strategy based on collective measurement of NPs, GDF-15, and galectin-3 (73,74).
Endothelial cell-derived micro vesicles and endothelial precursors
The endothelial dysfunction is established marker and direct player in nature evolution of HF. It is well known that severity of endothelial dysfunction independently associated with complications of HF and the risk of death due to HF-related events and CV death (73). The discoveries of novel biomarkers of endothelial dysfunction with high personalized significance led to determining brand new specific circulating biomarkers, such as endothelial micro vesicles (EMVs) and endothelial progenitor cells (EPCs) also known as endothelial precursors (73,74). Recent clinical studies have revealed that deficiency of circulating endothelial precursors were independent predictor of HF severity (74-76). Therefore, increased number of apoptotic MVs associated with decreased number activated endothelial cell-derived MVs predicted HF development and advance. This finding became to determine altered or impaired phenotype of MVs and now it is novel HF risk biomarker (77,78). Moreover, risk predictive score based on multiple biomarkers including impaired phenotype of MVs and deficiency of endothelial precursors was significantly superior to traditional biomarkers such as NPs, galectin-3, hs-CRP in HF risk stratification (78,79). However, it is not fully understood whether new HF predictive model would be more reliable in prognostication of HF treatment response. Large clinical trial is needed to clearly understand if personalized therapy of HF under new biomarker model control is better to traditional scores.
Biomarkers of collagen metabolism
Recent clinical studies have shown that impaired collagen metabolism may alter the myocardial collagen network and enable CV remodeling, and mediates HF complications, i.e., atrial fibrillation/flutter, sudden death, and decline LV pump function (80). Additionally, there are findings that BNP could influence on alterations of collagen type I metabolism in HF (77). The OPTIMAL (The Optimizing Congestive Heart Failure Outpatient Clinic trial) was revealed disturbances of collagen type I metabolism that are determined as independent predictor of long-term, all-cause CV mortality in HFrEF patients (81). Although these facts are limited and required to be elucidated carefully, it has been suggested that circulating CITP could be novel independent predictor of survival in HFrEF patients (82).
Matrix metalloproteinases (MMPs)
Development of HF strongly associates with CV remodeling, biomechanical and oxidative myocardial stress, neurohormonal and inflammatory activation that are modulated by MMPs. It has demonstrated that MMPs determine extracellular accumulation of collagen and mediate pro-fibrotic processes (82). Recent pre-clinical and clinical studies have revealed an altered expression of MMPs (MMP-1, MMP-3, MMP-6, MMP-9) and their tissue inhibitors was found in association with severity of cardiac dysfunction (82-86). Probably, the role of these biomarkers requires more future investigations to identify their ability to predict cardiac remodeling and HF-related outcomes.
Biomarkers of oxidative stress
Serum uric acid (SUA)
Recent studies have revealed the elevated level of SUA could be common feature for patients with numerous CV diseases including HF, hypertension, atherosclerosis, obesity, diabetes mellitus and chronic renal disease (87,88). The role of SUA in pathogenesis of HF is controversial. As strong oxidative factor uric acid triggers inflammation and frequently often impairs vascular function (89). In contrast, uric acid in peripheral tissues could act as scavenger of free radicals and protects against an oxidative stress (90,91). In routine clinical practice SUA remains a simple method for detection of HF risk at the early stage of HF advance (92-98). Moreover, serial measures of SUA levels are accurate predictor of clinical outcomes rather in acute HF than in chronic HF (95-97), while SUA undoubtedly remains a risk factor of poor outcomes in HF independently of LVEF (98,99).
Other biomarkers of oxidative stress
Serum levels of oxidative stress biomarkers (myeloperoxidase, vitamin D3, ceruloplasmin and 8-hydroxy-2'-deoxyguanosine) closely correlated with staging chronic HF regardless LVEF and predicted HFrEFm but not HFmrEF or HFpEF (70). Therefore, close link between vascular remodeling (98,99), endothelial dysfunction and CV disease the predictive role of vitamin signature in serum (i.e., vitamin A, B12, D, K, C and E) in HF individuals was found (99-102).
Biomarkers of renal dysfunction in HF
Cystatin C
Cystatin C was found an endogenous inhibitor of cysteine proteases. This biomarker is reported an alternative predictor of CV death in HF (95). The patients with HFrEF exhibited elevated serum levels of cystatin C in association with higher risk of HF-related complications (98,99), whereas in HFpEF patients this fact was not confirmed, while increased cystatin C level was found (100). Despite cystatin C has validated a predictor of kidney injury, the discriminative ability of this biomarker in chronic HF patients is lower to hs-CRP and NPs (99). In contrast, in acute HF individuals elevated cystatin C levels predicted poor prognosis better than NT-proBNP and SUA (103,104).
Other biomarkers of kidney injury in HF
There are promising biomarkers of kidney injury (stromal cell-derived factor-1, kidney injury molecule-1, exosomes, neutrophil gelatinase-associated lipocalin, interleukin-18) that recently had been used as prognosticators of HF (100,104). Because of all they are non-specific for HF, the implementation of them in routine practice is under fire and probably they could be incorporated into multiple biomarker models rather than single use in HF patients.
Genomic and epigenomic biomarkers
Genomic/epigenomic testing that now incorporated into diagnosis of inherited cardiomyopathies (105,106) appear to be promised method for HF risk stratification. Indeed, DNA methylation, ATP-dependent chromatin remodeling, histone modifications with an involvement of microRNA-related mechanisms are important pathophysiological factors contributing to adverse cardiac remodeling and altered cardiac function (107-110). There are numerous studies depicted the role of single nucleotide polymorphisms of genes encoding enzymes related to oxidative stress (111), genotype of guanine nucleotide-binding proteins beta-3 subunit (106), transcription factor Islet-1 gene (112,113), troponin T (114), CYP2D6 polymorphism (115), cardiac myosin binding protein-C mutations (116), renin-angiotensin-aldosterone system polymorphism (117), bradykinin type 1 receptor gene, angiotensin-II type I receptor gene, the β1-adrenoceptor gene and CYP2D6 polymorphism (118-120) in development of HF. However, encouraging results have not yet received (121-123), whereas controversial data are presented (124,125). Finally, the implementation of genomic/epigenomic markers into real clinical practice to predict HF development is so far future direction (126).
Micro-RNAs
It has been established that microRNAs (miRNA) are widely involved in the development and progression of HF across all pathophysiological stages of the disease (108). miRNA are epigenetic regulators of myocardial response and fibrosis, growth of cardiac myocytes, cardiac and vasculature reparation, immunity, angiogenesis, and inflammation (111). The altered miRNAs’ signature was found in patients with asymptomatic and symptomatic HF (127-129). It has suggested the signatures of non-coding RNAs would be candidate to improve diagnosis and prognostication of HF (130).
Mid-regional pro-adrenomedullin (ADM)
Mid-regional pro-adrenomedullin (MR-proADM) is the prohormone of the CV protein ADM and it is well-established neurohumoral marker of cardiac biochemical stress that was raised in patients with infections including sepsis, acute dyspnea, acute HF and severe chronic HFrEF/HFpEF, unstable angina pectoris/myocardial infarction, and throughout the first week after stroke (131). There is evidence regarding that the MR-proADM is an early predictor of in-hospital mortality due to various reasons, i.e. respiratory infections, surgical procedure and CV diseases (132-134). MR-proADM as a marker of biomechanical stress and fibrosis was not better than NPs and did not exhibit equal predictive value to sST2r and galectin-3 in HFrEF/HFpEF (134). Interestingly, sST2 was better to MR-proADM, because it is more closely related to LV remodeling and cardiac fibrosis. Moreover, MR-proADM did not improve a risk stratification based on NPs in patients with chronic HFrEF and moderate anaemia (135). Thus, the role of MR-proADM as a component of biomarker-based stratification is discussable, while the biomarker can contribute to determine the short-term outcomes of critical ill patients with acute severe dyspnea, respiratory infection and acute HF.
Validation of multiple biomarker predictive scores
There are numerous biomarker predictive scores that have approved for chronic HF, whereas predictive models for acute HF have not yet validated (136,137). Current multiple biomarker scores affecting prognostication, risk stratification and diagnosis of HF (Figure 2) are based on NPs in combination with biomarkers of myocardial injury and fibrosis (galectin-3 and sST2 receptor). A new score validated by the American Heart Association/American College of Cardiology [2017] is fitted for patients at risk of HF, with established chronic HF (for HFrEF and HFpEF, but not for HFmrEF), with suspected and documented acute HF (at admission), as well as patients with HF at discharge from the hospital.
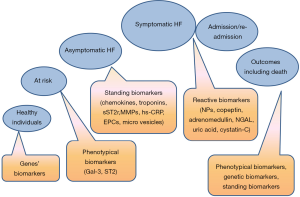
Interestingly, there are several attempts regarding use of biomarkers to stratify at risk patients with different phenotypes of HF, such as HFrEF, HFmrEF or HFpEF. Whether add-on biomarkers to based models are needed to improve cumulative predictive value for wide circle of HF individuals with different HF phenotypes, co-morbidities, ages and sex-related particularities is not fully elucidated. It has suggested that sST2 and galectin-3 might sufficiently improve prognosis in HF-related hospitalization and CV death, when they had added to NPs. This strategy is confirmed by experts of various medical associations and the only one is validated now.
Conclusions
There are several controversies regarding the importance of biomarkers as predictors of survival and in diagnosis of HF. Improvement of clinical guidelines for optimizing HF therapy in routine clinical practice under biomarkers’ control is required. Obviously, galectin-3 or sST2 would be optimal for improving NPs-based biomarker strategy in HF individuals, while there is large body of evidence that other biomarkers could individualize a risk stratification and predict treatment response. There is need of larger clinical trials in order to direct compare different biomarkers and clarify their role in diagnosis and guided therapy of HF.
Acknowledgments
Funding: None.
Footnote
Provenance and Peer Review: This article was commissioned by the Guest Editor (Fabian Sanchis-Gomar) for the series “Biomarkers in cardiovascular disease” published in Journal of Laboratory and Precision Medicine. The article has undergone external peer review.
Conflicts of Interest: The author has completed the ICMJE uniform disclosure form (available at http://dx.doi.org/10.21037/jlpm.2018.03.13). The series “Biomarkers in Cardiovascular Disease” was commissioned by the editorial office without any funding or sponsorship. The author has no other conflicts of interest to declare.
Ethical Statement: The author is accountable for all aspects of the work in ensuring that questions related to the accuracy or integrity of any part of the work are appropriately investigated and resolved.
Open Access Statement: This is an Open Access article distributed in accordance with the Creative Commons Attribution-NonCommercial-NoDerivs 4.0 International License (CC BY-NC-ND 4.0), which permits the non-commercial replication and distribution of the article with the strict proviso that no changes or edits are made and the original work is properly cited (including links to both the formal publication through the relevant DOI and the license). See: https://creativecommons.org/licenses/by-nc-nd/4.0/.
References
- Ponikowski P, Voors AA, Anker SD, et al. Authors/Task Force Members. 2016 ESC guidelines for the diagnosis and treatment of acute and chronic heart failure: the Task Force for the diagnosis and treatment of acute and chronic heart failure of the European Society of Cardiology (ESC) developed with the special contribution of the Heart Failure Association (HFA) of the ESC. Eur Heart J 2016;37:2129-200. [Crossref] [PubMed]
- Timmermans I, Denollet J, Pedersen SS, et al. Patient-reported causes of heart failure in a large European sample. Int J Cardiol 2018;258:179-84. [Crossref] [PubMed]
- Aimo A, Vergaro G, Barison A, et al. Sex-related differences in chronic heart failure. Int J Cardiol 2018;255:145-51. [Crossref] [PubMed]
- Wettersten N, Maisel AS. Biomarkers for Heart Failure: An Update for Practitioners of Internal Medicine. Am J Med 2016;129:560-7. [Crossref] [PubMed]
- Ledwidge M, Gallagher J, Conlon C, et al. Natriuretic peptide-based screening and collaborative care for heart failure: the STOP-HF randomized trial. JAMA 2013;310:66-74. [Crossref] [PubMed]
- Biomarkers Definitions Working Group (National Institutes of Health). Biomarkers and surrogate endpoints: preferred definitions and conceptual framework. Clin Pharmacol Ther 2001;69:89-95. [Crossref] [PubMed]
- Mouhieddine TH, El Houjeiri L, Sabra M, et al. Chapter 20: CNS Trauma Biomarkers and Surrogate Endpoints Pipeline from Bench to Bedside: A Translational Perspective. In: Kobeissy FH. editor. Brain Neurotrauma: Molecular, Neuropsychological, and Rehabilitation Aspects. Boca Raton (FL): CRC Press/Taylor & Francis, 2015.
- Berezin AE. Contemporary approaches of biological markers in heart failure/Scholars' Press, Omni Scriptum Management GmbH, Saarbrücken, Germany, 2017.
- Doumouras BS, Lee DS, Levy WC, et al. An appraisal of biomarker-based risk-scoring models in chronic heart failure: which one is best? Curr Heart Fail Rep 2018;15:24-36. [Crossref] [PubMed]
- Chow SL, Maisel AS, Anand I, et al. Role of Biomarkers for the Prevention, Assessment, and Management of Heart Failure: A Scientific Statement From the American Heart Association. Circulation 2017;135:e1054-e1091. [Crossref] [PubMed]
- Kosmala W, Przewlocka-Kosmala M, Rojek A, et al. Comparison of the diastolic stress test with a combined resting echocardiography and biomarker approach to patients with exertional dyspnea: diagnostic and prognostic implications. JACC Cardiovasc Imaging 2018; [Epub ahead of print]. [Crossref] [PubMed]
- Fonarow GC. Biomarker-Guided vs Guideline-Directed Titration of Medical Therapy for Heart Failure. JAMA 2017;318:707-8. [Crossref] [PubMed]
- Vodovar N, Mebazaa A, Januzzi JL Jr, et al. Evolution of natriuretic peptide biomarkers in heart failure: Implications for clinical care and clinical trials. Int J Cardiol 2018;254:215-21. [Crossref] [PubMed]
- Felker GM, Anstrom KJ, Adams KF, et al. Effect of Natriuretic Peptide-Guided Therapy on Hospitalization or Cardiovascular Mortality in High-Risk Patients With Heart Failure and Reduced Ejection Fraction: A Randomized Clinical Trial. JAMA 2017;318:713-20. [Crossref] [PubMed]
- Bayes-Genis A, de Antonio M, Vila J, et al. Head-to-head comparison of 2 myocardial fibrosis biomarkers for long-term heart failure risk stratification: ST2 versus galectin-3. J Am Coll Cardiol 2014;63:158-66. [Crossref] [PubMed]
- Anguita M. High-sensitivity troponins and prognosis of heart failure. Rev Clin Esp 2017;217:95-6. [Crossref] [PubMed]
- Billebeau G, Vodovar N, Sadoune M, et al. Effects of a cardiac rehabilitation programme on plasma cardiac biomarkers in patients with chronic heart failure. Eur J Prev Cardiol 2017;24:1127-35. [Crossref] [PubMed]
- Imran TF, Shin HJ, Mathenge N, et al. Meta-Analysis of the Usefulness of Plasma Galectin-3 to Predict the Risk of Mortality in Patients With Heart Failure and in the General Population. Am J Cardiol 2017;119:57-64. [Crossref] [PubMed]
- Boulogne M, Sadoune M, Launay JM, et al. Inflammation versus mechanical stretch biomarkers over time in acutely decompensated heart failure with reduced ejection fraction. Int J Cardiol 2017;226:53-9. [Crossref] [PubMed]
- de Boer RA, Nayor M, deFilippi CR, et al. Association of Cardiovascular Biomarkers With Incident Heart Failure With Preserved and Reduced Ejection Fraction. JAMA Cardiol 2018;3:215-24. [Crossref] [PubMed]
- Souza BSF, Silva DN, Carvalho RH, et al. Association of Cardiac Galectin-3 Expression, Myocarditis, and Fibrosis in Chronic Chagas Disease Cardiomyopathy. Am J Pathol 2017;187:1134-46. [Crossref] [PubMed]
- Di Tano G, Caretta G, De Maria R, et al. Galectin-3 and outcomes after anterior-wall myocardial infarction treated by primary percutaneous coronary intervention. Biomark Med 2018;12:21-6. [Crossref] [PubMed]
- Binas D, Daniel H, Richter A, et al. The prognostic value of sST2 and galectin-3 considering different aetiologies in non-ischaemic heart failure. Open Heart 2018;5:e000750 [Crossref] [PubMed]
- van Vark LC, Lesman-Leegte I, Baart SJ, et al. Prognostic Value of Serial Galectin-3 Measurements in Patients With Acute Heart Failure. J Am Heart Assoc. 2017;6: [Crossref] [PubMed]
- Srivatsan V, George M, Shanmugam E. Utility of galectin-3 as a prognostic biomarker in heart failure: where do we stand? Eur J Prev Cardiol 2015;22:1096-110. [Crossref] [PubMed]
- Feng SD, Jiang Y, Lin ZH, et al. Diagnostic value of brain natriuretic peptide and β-endorphin plasma concentration changes in patients with acute left heart failure and atrial fibrillation. Medicine (Baltimore) 2017;96:e7526 [Crossref] [PubMed]
- Yancy CW, Jessup M, Bozkurt B, et al. 2017 ACC/AHA/HFSA Focused Update of the 2013 ACCF/AHA Guideline for the Management of Heart Failure: A Report of the American College of Cardiology/American Heart Association Task Force on Clinical Practice Guidelines and the Heart Failure Society of America. J Card Fail 2017;23:628-51. [Crossref] [PubMed]
- Lala RI, Lungeanu D, Darabantiu D, et al. Galectin-3 as a marker for clinical prognosis and cardiac remodeling in acute heart failure. Herz 2018;43:146-55. [Crossref] [PubMed]
- Nagarajan V, Hernandez AV, Tang WH. Prognostic value of cardiac troponin in chronic stable heart failure: a systematic review. Heart 2012;98:1778-86. [Crossref] [PubMed]
- Masson S, Latini R, Anand IS. An update on cardiac troponins as circulating biomarkers in heart failure. Curr Heart Fail Rep 2010;7:15-21. [Crossref] [PubMed]
- Malek V, Gaikwad AB. Neprilysin inhibitors: A new hope to halt the diabetic cardiovascular and renal complications? Biomed Pharmacother 2017;90:752-9. [Crossref] [PubMed]
- Wong PC, Guo J, Zhang A. The renal and cardiovascular effects of natriuretic peptides. Absence of clear clinical recommendations of biomarker-based HF therapy is the main cause of uncertainty regarding practical use of this approach. Adv Physiol Educ 2017;41:179-85. [Crossref] [PubMed]
- Luchner A, von Haehling S, Holubarsch C, et al. Indications and Clinical Implications of the Use of the Cardiac Markers BNP and NT-proBNP. Dtsch Med Wochenschr 2017;142:346-55. [PubMed]
- Aspromonte N, Gulizia MM, Clerico A, et al. ANMCO/ELAS/SIBioC Consensus document: Recommendations for the use of cardiac biomarkers in heart failure patients). G Ital Cardiol (Rome) 2016;17:615-56. [PubMed]
- Skaf S, Thibault B, Khairy P, et al. Impact of Left Ventricular vs Biventricular Pacing on Reverse Remodelling: Insights From the Evaluation of Resynchronization Therapy for Heart Failure (EARTH) Trial. Can J Cardiol 2017;33:1274-82. [Crossref] [PubMed]
- Nakanishi M, Nakao K, Kumasaka L, et al. Improvement in Exercise Capacity by Exercise Training Associated With Favorable Clinical Outcomes in Advanced Heart Failure With High B-Type Natriuretic Peptide Level. Circ J 2017;81:1307-14. [Crossref] [PubMed]
- Nymo SH, Aukrust P, Kjekshus J, et al. Limited Added Value of Circulating Inflammatory Biomarkers in Chronic Heart Failure. JACC Heart Fail 2017;5:256-64. [Crossref] [PubMed]
- Berezin AE. Prognostication in different heart failure phenotypes: the role of circulating biomarkers. J Circ Biomark 2016;5:01.
- Favresse J, Gruson D. Natriuretic peptides: degradation, circulating forms, dosages and new therapeutic approaches. Ann Biol Clin (Paris) 2017;75:259-67. [PubMed]
- Besler C, Lang D, Urban D, et al. Plasma and Cardiac Galectin-3 in Patients With Heart Failure Reflects Both Inflammation and Fibrosis: Implications for Its Use as a Biomarker. Circ Heart Fail 2017;10: [Crossref] [PubMed]
- Krintus M, Kozinski M, Fabiszak T, et al. Establishing reference intervals for galectin-3 concentrations in serum requires careful consideration of its biological determinants. Clin Biochem 2017;50:599-604. [Crossref] [PubMed]
- Meijers WC, van der Velde AR, Muller Kobold AC, et al. Variability of biomarkers in patients with chronic heart failure and healthy controls. Eur J Heart Fail 2017;19:357-65. [Crossref] [PubMed]
- Agnello L, Bivona G, Sasso BL, et al. Galectin-3 in acute coronary syndrome. Clin Biochem 2017;50:797-803. [Crossref] [PubMed]
- Miró Ò, González de la Presa B, Herrero-Puente P, et al. The GALA study: relationship between galectin-3 serum levels and short- and long-term outcomes of patients with acute heart failure. Biomarkers 2017;22:731-9. [Crossref] [PubMed]
- Wojciechowska C, Romuk E, Nowalany-Kozielska E, et al. Serum Galectin-3 and ST2 as predictors of unfavorable outcome in stable dilated cardiomyopathy patients. Hellenic J Cardiol 2017;58:350-9. [Crossref] [PubMed]
- Maisel AS, Di Somma S. Do we need another heart failure biomarker: focus on soluble suppression of tumorigenicity 2 (sST2). Eur Heart J 2017;38:2325-33. [PubMed]
- Berezin AE. Biomarkers for cardiovascular risk in diabetic patients. Heart 2016;102:1939-41. [Crossref] [PubMed]
- AbouEzzeddine OF, McKie PM, Dunlay SM, et al. Suppression of tumorigenicity 2 in heart failure with preserved ejection fraction. J Am Heart Assoc 2017;6: [Crossref] [PubMed]
- Aimo A, Vergaro G, Ripoli A, et al. Meta-Analysis of Soluble Suppression of Tumorigenicity-2 and Prognosis in Acute Heart Failure. JACC Heart Fail 2017;5:287-96. [Crossref] [PubMed]
- Berezin AE, Kremzer AA, Martovitskaya YV, et al. The novel biomarker risk prediction score in patients with chronic heart failure. Clin Hypertens 2016;22:3. [Crossref] [PubMed]
- Pouleur AC. Which biomarkers do clinicians need for diagnosis and management of heart failure with reduced ejection fraction? Clin Chim Acta 2015;443:9-16. [Crossref] [PubMed]
- Ryu JA, Yang JH, Lee D, et al. Clinical Usefulness of Procalcitonin and C-Reactive Protein as Outcome Predictors in Critically Ill Patients with Severe Sepsis and Septic Shock. PloS One 2015;10:e0138150 [Crossref] [PubMed]
- Reiner MM, Khoury WE, Canales MB, et al. Procalcitonin as a biomarker for predicting amputation level in lower extremity infections. J Foot Ankle Surg 2017;56:484-91. [Crossref] [PubMed]
- Hayashida K, Kondo Y, Hara Y, et al. Head-to-head comparison of procalcitonin and presepsin for the diagnosis of sepsis in critically ill adult patients: a protocol for a systematic review and meta-analysis. BMJ Open 2017;7:e014305 [Crossref] [PubMed]
- Simon L, Gauvin F, Amre DK, et al. Serum procalcitonin and C-reactive protein levels as markers of bacterial infection: a systematic review and meta-analysis. Clin Infect Dis 2004;39:206-17. [Crossref] [PubMed]
- Morgenthaler NG. Assay for the measurement of copeptin, a stable peptide derived from the precursor of vasopressin. Clin Chem 2006;52:112-9. [Crossref] [PubMed]
- Remde H, Dietz A, Emeny R, et al. The cardiovascular markers copeptin and high-sensitive C-reactive protein decrease following specific therapy for primary aldosteronism. J Hypertens 2016;34:2066-73. [Crossref] [PubMed]
- Moayedi Y, Ross HJ. Advances in heart failure: a review of biomarkers, emerging pharmacological therapies, durable mechanical support and telemonitoring. Clin Sci (Lond) 2017;131:553-66. [Crossref] [PubMed]
- Krane V, Genser B, Kleber ME, et al. Copeptin associates with cause-specific mortality in patients with impaired renal function: results from the LURIC and the 4D Study. Clin Chem 2017;63:997-1007. [Crossref] [PubMed]
- Yan JJ, Lu Y, Kuai ZP, et al. Predictive value of plasma copeptin level for the risk and mortality of heart failure: a meta-analysis. J Cell Mol Med 2017;21:1815-25. [Crossref] [PubMed]
- Berezin AE. Biological markers of cardiovascular diseases. Part 4. Diagnostic and prognostic value of biological markers at risk stratification among patients with heart failure. Moskow: LAMBERT Academic Publishing GmbH, 2015:329.
- Savic-Radojevic A, Pljesa-Ercegovac M, Matic M, et al. Novel Biomarkers of Heart Failure. Adv Clin Chem 2017;79:93-152. [Crossref] [PubMed]
- Smaradottir MI, Ritsinger V, Gyberg V, et al. Copeptin in patients with acute myocardial infarction and newly detected glucose abnormalities - A marker of increased stress susceptibility? A report from the Glucose in Acute Myocardial Infarction cohort. Diab Vasc Dis Res 2017;14:69-76. [Crossref] [PubMed]
- Sahin I, Gungor B, Ozkaynak B, et al. Higher copeptin levels are associated with worse outcome in patients with hypertrophic cardiomyopathy. Clin Cardiol 2017;40:32-7. [Crossref] [PubMed]
- Herrero-Puente P, Prieto-García B, García-García M, et al. Predictive capacity of a multimarker strategy to determine short-term mortality in patients attending a hospital emergency Department for acute heart failure. BIO-EAHFE study. Clin Chim Acta 2017;466:22-30. [Crossref] [PubMed]
- Kitai T, Kim YH, Kiefer K, et al. Circulating intestinal fatty acid-binding protein (I-FABP) levels in acute decompensated heart failure. Clin Biochem 2017;50:491-5. [Crossref] [PubMed]
- Chmurzyńska A. The multigene family of fatty acidbinding proteins (FABPs): function, structure, and polymorphism. J Appl Genet 2006;47:39-48. [Crossref] [PubMed]
- Qian HY, Huang J, Yang YJ, et al. Heart-type Fatty Acid Binding Protein in the Assessment of Acute Pulmonary Embolism. Am J Med Sci 2016;352:557-62. [Crossref] [PubMed]
- Kempf T, Wollert KC. Growth differentiation factor-15: a new biomarker in cardiovascular disease. Herz 2009;34:594-9. [Crossref] [PubMed]
- Chan MM, Santhanakrishnan R, Chong JP, et al. Growth differentiation factor 15 in heart failure with preserved vs. reduced ejection fraction. Eur J Heart Fail 2016;18:81-8. [Crossref] [PubMed]
- Hage C, Michaëlsson E, Linde C, et al. Inflammatory biomarkers predict heart failure severity and prognosis in patients with heart failure with preserved ejection fraction: a holistic proteomic approach. Circ Cardiovasc Genet 2017;10: [Crossref] [PubMed]
- Demissei BG, Cotter G, Prescott MF, et al. A multimarker multi-time point-based risk stratification strategy in acute heart failure: results from the RELAX-AHF trial. Eur J Heart Fail 2017;19:1001-10. [Crossref] [PubMed]
- Berezin AE, Kremzer AA, Martovitskaya YV, et al. The utility of biomarker risk prediction score in patients with chronic heart failure. Int J Clin Exp Med 2015;8:18255-64. [PubMed]
- Berezin AE. The risk stratification in heart failure patients: The controversial role of high-sensitive ST2. J Integr Cardiol 2015;1:216-7.
- Berezin A. Endothelial Derived Micro Particles: Biomarkers for Heart Failure Diagnosis and Management. J Clin Trial Cardiol 2015;2:1-3. [Crossref]
- Berezin AE, Kremzer AA, Samura TA, et al. Apoptotic microparticles to progenitor mononuclear cells ratio in heart failure: relevance of clinical status and outcomes. JCvD 2014;2:50-7.
- Berezin AE. Impaired Phenotype of Endothelial Cell-Derived Micro Particles: The Missed Link in Heart Failure Development? Biomarkers J 2016;2:6.
- Berezin AE, Kremzer AA, Berezina TA, et al. Pattern of circulating microparticles in chronic heart failure patients with metabolic syndrome: relevance to neurohumoral and inflammatory activation. BBA Clin 2015;4:69-75. [Crossref] [PubMed]
- Berezin AE. Impaired pattern of endothelial derived microparticles in heart failure patients. J Mol Genet Med 2015;9:152.
- Löfsjögård J, Persson H, Díez J, et al. Atrial fibrillation and biomarkers of myocardial fibrosis in heart failure. Scand Cardiovasc J 2014;48:299-303. [Crossref] [PubMed]
- Löfsjögård J, Kahan T, Díez J, et al. Usefulness of Collagen Carboxy-Terminal Propeptide and Telopeptide to Predict Disturbances of Long-Term Mortality in Patients ≥60 Years With Heart Failure and Reduced Ejection Fraction. Am J Cardiol 2017;119:2042-8. [Crossref] [PubMed]
- Tziakas DN, Chalikias GK, Stakos D, et al. Independent and additive prognostic ability of serum carboxy-terminal telopeptide of collagen type-I in heart failure patients: a multi-marker approach with high-negative predictive value to rule out long-term adverse events. Eur J Prev Cardiol 2012;19:62-71. [Crossref] [PubMed]
- Berezin AE, Samura TA. Prognostic value of biological markers in myocardial infarction patients. Asian Cardiovasc Thorac Ann 2013;21:142-50. [Crossref] [PubMed]
- Collier P, Watson CJ, Voon V, et al. Can emerging biomarkers of myocardial emodeling identify asymptomatic hypertensive patients at risk for diastolic dysfunction and diastolic heart failure? Eur J Heart Fail 2011;13:1087-95. [Crossref] [PubMed]
- Hutchinson KR, Stewart JA Jr, Lucchesi PA. Extracellular matrix remodeling during the progression of volume overload-induced heart failure. J Mol Cell Cardiol 2010;48:564-9. [Crossref] [PubMed]
- Berezin AE, Kremzer AA, Samura TA. Circulating thrombospondine-2 in patients with moderate-to-severe chronic heart failure due to coronary artery disease. J Biomed Res 2015;30. [Epub ahead of print]. [PubMed]
- Grassi D, Ferri L, Desideri G, et al. Chronic hyperuricemia, uric acid deposit and cardiovascular risk. Curr Pharm Des 2013;19:2432-8. [Crossref] [PubMed]
- Borghi C, Rosei EA, Bardin T, et al. Serum uric acid and the risk of cardiovascular and renal disease. J Hypertens 2015;33:1729-41. [Crossref] [PubMed]
- Berezin AE, Kremzer AA. Serum uric acid as a marker of coronary calcification in patients with asymptomatic coronary artery disease with preserved left ventricular pump function. Cardiol Res Pract 2013;2013:129369 [PubMed]
- Berezin AE. Serum uric acid as a metabolic regulator of endothelial reparative processes in heart failure patients. Stem Cell & Translational Investigation 2014;1:e432
- Berezin AE, Kremzer AA, Martovitskaya YV, et al. Serum Uric Acid Predicts Declining of Circulating Proangiogenic Mononuclear Progenitor Cells in Chronic Heart Failure Patients. J Cardiovasc Thorac Res 2014;6:153-62. [Crossref] [PubMed]
- Amin A, Chitsazan M, Shiukhi Ahmad Abad F, et al. On admission serum sodium and uric acid levels predict 30 day rehospitalization or death in patients with acute decompensated heart failure. ESC Heart Fail 2017;4:162-8. [Crossref] [PubMed]
- Okazaki H, Shirakabe A, Kobayashi N, et al. The prognostic impact of uric acid in patients with severely decompensated acute heart failure. J Cardiol 2016;68:384-91. [Crossref] [PubMed]
- Okazaki H, Shirakabe A, Kobayashi N, et al. Are atherosclerotic risk factors associated with a poor prognosis in patients with hyperuricemic acute heart failure? The evaluation of the causal dependence of acute heart failure and hyperuricemia. Heart Vessels 2017;32:436-45. [Crossref] [PubMed]
- Otaki Y, Watanabe T, Kinoshita D, et al. Association of plasma xanthine oxidoreductase activity with severity and clinical outcome in patients with chronic heart failure. Int J Cardiol 2017;228:151-7. [Crossref] [PubMed]
- Huerta A, López B, Ravassa S, et al. Association of cystatin C with heart failure with preserved ejection fraction in elderly hypertensive patients: potential role of altered collagen metabolism. J Hypertens 2016;34:130-8. [Crossref] [PubMed]
- Kim H, Yoon HJ, Park HS, et al. Potentials of cystatin C and uric acid for predicting prognosis of heart failure. Congest Heart Fail 2013;19:123-9. [Crossref] [PubMed]
- Berezin A. Does serum uric acid play a protective role against tissue damage in cardiovascular and metabolic diseases? Ann Clin Hypertens 2017;1:39-41. [Crossref]
- Berezin A. Biomarkers in heart failure. J Blood Lymph 2017;7:172-9.
- Berezin A. Up-to-date clinical approaches of biomarkers’ use in heart failure. Biomed Res Ther 2017;4:1341-70. [Crossref]
- Mozos I, Marginean O. Links between vitamin D deficiency and cardiovascular diseases. BioMed Res Int 2015;2015:109275 [PubMed]
- Mozos I, Stoian D, Luca CT. Crosstalk between vitamin A, B12, D, K, C and E status and arterial stiffness. Dis Markers 2017;2017:8784971 [PubMed]
- Kim TH, Kim H, Kim IC. The potential of cystatin-C to evaluate the prognosis of acute heart failure: A comparative study. Acute Card Care 2015;17:72-6. [Crossref] [PubMed]
- Taub PR, Borden KC, Fard A, et al. Role of biomarkers in the diagnosis and prognosis of acute kidney injury in patients with cardiorenal syndrome. Expert Rev Cardiovasc Ther 2012;10:657-67. [Crossref] [PubMed]
- Teekakirikul P, Kelly MA, Rehm HL, et al. Inherited cardiomyopathies: molecular genetics and clinical genetic testing in the postgenomic era. J Mol Diagn 2013;15:158-70. [Crossref] [PubMed]
- Teo LY, Moran RT, Tang WH. Evolving Approaches to Genetic Evaluation of Specific Cardiomyopathies. Curr Heart Fail Rep 2015;12:339-49. [Crossref] [PubMed]
- Hershberger RE, Siegfried JD. Update 2011: clinical and genetic issues in familial dilated cardiomyopathy. J Am Coll Cardiol 2011;57:1641-9. [Crossref] [PubMed]
- Berezin A. Epigenetics in heart failure phenotypes. BBA Clinical 2016;6:31-7. [Crossref] [PubMed]
- Yang J, Xu WW, Hu SJ. Heart failure: advanced development in genetics and epigenetics. Biomed Res Int 2015;2015:352734 [PubMed]
- Lopes LR, Elliott PM. Genetics of heart failure. Biochim Biophys Acta 2013;1832:2451-61. [Crossref] [PubMed]
- Berezin AE. Epigenetically Modified Endothelial Progenitor Cells in Heart Failure. J Clin Epigenet 2016;2:13. [PubMed]
- Fazakas Á, Szelényi Z, Szénási G, et al. Genetic predisposition in patients with hypertension and normal ejection fraction to oxidative stress. J Am Soc Hypertens 2016;10:124-32. [Crossref] [PubMed]
- McNamara DM, Taylor AL, Tam SW, et al. G-protein beta-3 subunit genotype predicts enhanced benefit of fixed-dose isosorbide dinitrate and hydralazine: results of A-HeFT. JACC Heart Fail 2014;2:551-7. [Crossref] [PubMed]
- Friedrich FW, Dilanian G, Khattar P, et al. A novel genetic variant in the transcription factor Islet-1 exerts gain of function on myocyte enhancer factor 2C promoter activity. Eur J Heart Fail 2013;15:267-76. [Crossref] [PubMed]
- Hofman N, van Langen I, Wilde AAM. Genetic testing in cardiovascular diseases. Curr Opin Cardiol 2010;25:243-8. [Crossref] [PubMed]
- Sutter ME, Gaedigk A, Albertson TE, et al. Polymorphisms in CYP2D6 may predict methamphetamine related heart failure. Clin Toxicol (Phila) 2013;51:540-4. [Crossref] [PubMed]
- Kolder IC, Michels M, Christiaans I, et al. The role of renin-angiotensin-aldosterone system polymorphisms in phenotypic expression of MYBPC3-related hypertrophic cardiomyopathy. Eur J Hum Genet 2012;20:1071-7. [Crossref] [PubMed]
- Wu CK, Luo JL, Tsai CT, et al. Demonstrating the pharmacogenetic effects of angiotensin-converting enzyme inhibitors on long-term prognosis of diastolic heart failure. Pharmacogenomics J 2010;10:46-53. [Crossref] [PubMed]
- Wu CK, Luo JL, Wu XM, et al. A propensity score-based case-control study of renin-angiotensin system gene polymorphisms and diastolic heart failure. Atherosclerosis 2009;205:497-502. [Crossref] [PubMed]
- Yu B, Zheng Y, Alexander D, Manolio TA, et al. Genome-wide association study of a heart failure related metabolomic profile among African Americans in the Atherosclerosis Risk in Communities (ARIC) study. Genet Epidemiol 2013;37:840-5. [Crossref] [PubMed]
- Ganna A, Rivadeneira F, Hofman A, et al. Genetic determinants of mortality. Can findings from genome-wide association studies explain variation in human mortality? Hum Genet 2013;132:553-61. [Crossref] [PubMed]
- Yip VL, Pirmohamed M. Expanding role of pharmacogenomics in the management of cardiovascular disorders. Am J Cardiovasc Drugs 2013;13:151-62. [Crossref] [PubMed]
- Nelveg-Kristensen KE, Busk Madsen M, Torp-Pedersen C, et al. Pharmacogenetic Risk Stratification in Angiotensin-Converting Enzyme Inhibitor-Treated Patients with Congestive Heart Failure: A Retrospective Cohort Study. PLoS One 2015;10:e0144195 [Crossref] [PubMed]
- Bondar G, Cadeiras M, Wisniewski N, et al. Comparison of whole blood and peripheral blood mononuclear cell gene expression for evaluation of the perioperative inflammatory response in patients with advanced heart failure. PLoS One 2014;9:e115097 [Crossref] [PubMed]
- Berezin AE. Genetic Predictive Scores in Heart Failure: Possibilities and Expectations. J Data Mining Genomics Proteomics 2016;7:e127 [Crossref]
- Poller W, Dimmeler S, Heymans S, et al. Non-coding RNAs in cardiovascular diseases: diagnostic and therapeutic perspectives. Eur Heart J 2017; [Epub ahead of print]. [Crossref] [PubMed]
- Jin P, Gu W, Lai Y, et al. The Circulating MicroRNA-206 Level Predicts the Severity of Pulmonary Hypertension in Patients with Left Heart Diseases. Cell Physiol Biochem 2017;41:2150-60. [Crossref] [PubMed]
- Vegter EL, van der Meer P, Voors AA. Associations between volume status and circulating microRNAs in acute heart failure. Eur J Heart Fail 2017; [Epub ahead of print]. [Crossref] [PubMed]
- Yan H, Ma F, Zhang Y, et al. miRNAs as biomarkers for diagnosis of heart failure: A systematic review and meta-analysis. Medicine (Baltimore) 2017;96:e6825 [Crossref] [PubMed]
- Wong CM, Hawkins NM, Petrie MC, et al. Heart failure in younger patients: the Meta-analysis Global Group in Chronic Heart Failure (MAGGIC). Eur Heart J 2014;35:2714-21. [Crossref] [PubMed]
- Bustamante A, García-Berrocoso T, Penalba A, et al. Sepsis biomarkers reprofiling to predict stroke-associated infections. J Neuroimmunol 2017;312:19-23. [Crossref] [PubMed]
- Odermatt J, Meili M, Hersberger L, et al. Pro-Adrenomedullin predicts 10-year all-cause mortality in community-dwelling patients: a prospective cohort study. BMC Cardiovasc Disord 2017;17:178. [Crossref] [PubMed]
- Dres M, Hausfater P, Foissac F, et al. Mid-regional pro-adrenomedullin and copeptin to predict short-term prognosis of COPD exacerbations: a multicenter prospective blinded study. Int J Chron Obstruct Pulmon Dis 2017;12:1047-56. [Crossref] [PubMed]
- Lopes D, Menezes Falcão L. Mid-regional pro-adrenomedullin and ST2 in heart failure: Contributions to diagnosis and prognosis. Rev Port Cardiol 2017;36:465-72. [Crossref] [PubMed]
- Welsh P, Kou L, Yu C, et al. Prognostic importance of emerging cardiac, inflammatory, and renal biomarkers in chronic heart failure patients with reduced ejection fraction and anaemia: RED-HF study. Eur J Heart Fail 2018;20:268-77. [Crossref] [PubMed]
- Bayes-Genis A, Ordonez-Llanos J. Multiple biomarker strategies for risk stratification in heart failure. Clin Chim Acta 2015;443:120-5. [Crossref] [PubMed]
- Cohen-Solal A, Laribi S, Ishihara S, et al. Prognostic markers of acute decompensated heart failure: the emerging roles of cardiac biomarkers and prognostic scores. Arch Cardiovasc Dis 2015;108:64-74. [Crossref] [PubMed]
Cite this article as: Berezin AE. Circulating biomarkers in heart failure: diagnostic and prognostic importance. J Lab Precis Med 2018;3:36.