GlycA: a new biomarker for systemic inflammation and cardiovascular disease (CVD) risk assessment
Inflammation as a key driver of cardiovascular disease (CVD)
Although there was resistance when the concept was first proposed in the early 1990s, it is now widely accepted that inflammation plays a pivotal role in the pathogenesis of atherosclerosis (1-3). The actual sequence of events and initial triggers of the inflammatory cascade underlying atherosclerosis remain an active area of investigation. However, it is widely accepted that it starts with some type of endothelial injury, which allows for LDL and small remnant lipoproteins to across the endothelium, infiltrating into the intima. Within the intimal space, these lipoproteins are subject to various modifications, such as aggregation and oxidation, triggering monocyte recruitment, which subsequently enter the intimal space and begin the phagocytosis of the variously modified lipoprotein particles trapped in there (3,4). This process eventually becomes self-propagating, inducing further damage to the overlying endothelium, which in turn allows for more lipoprotein infiltration and macrophage recruitment and activation. At some point, the ongoing inflammation also triggers the recruitment of T-lymphocytes, which further enhance the inflammatory response by secreting various cytokines that stimulate the proliferation of, and extracellular matrix deposition by the underlying vascular smooth muscle cells (3-5). Thus, it is now commonplace to consider atherosclerosis a chronic low-grade inflammatory process that starts many years before atherosclerotic plaque becomes visible by current imaging modalities (3-5). It is worth mentioning that the N-glycosylation patterns of several membrane proteins localized to the apical surface of endothelial cells also undergo extensive modifications in the setting of inflammation, some of which appear to play important roles in mediating the binding, activation, and migration of leukocytes (6). Additionally, recent studies have shown that reduced N-glycosylation, such as would be seen in inherited defects of glycosylation, result in reduced apolipoprotein B (apoB) and LDL-C levels by inducing increased LDL receptor expression (7). This clearly suggests that alterations in the glycosylation patterns of circulating or endothelial-bound proteins, may also contribute to the atherogenesis process.
Among the major landmark studies supporting the inflammatory hypothesis of atherosclerosis was the recent CANTOS trial, which demonstrated for the first time that dampening the baseline inflammatory response in patients with a prior CVD event or elevated baseline high-sensitivity C-reactive protein (hsCRP), using Canakinumab, a monoclonal antibody targeting IL-1-beta, leads to a significant reduction in the rates of recurrent CVD events (8). As a consequence, there is now great interest in identifying safe and inexpensive anti-inflammatory agents that can alleviate the inflammatory response underlying CVD development, such as colchicine or methotrexate (5,9,10). These studies also highlight the importance of identifying inflammatory biomarkers having a predictive value for CVD risk assessment, so that they can be used in monitoring the therapeutic response to the various anti-inflammatory agents under investigation for CVD risk reduction (11,12). One such candidate is hsCRP, a routinely tested inflammatory marker with good analytical performance (13), which has been consistently shown to positively correlate with future CVD events (1,13-15). Several other proteins related to the inflammatory cascade have also been suggested as possible biomarkers for CVD risk assessment, albeit having a weaker supporting evidence. These include myeloperoxidase (MPO), fibrinogen, interleukin-6 (IL-6), lipoprotein-associated phospholipase A2 (Lp-PLA2), and serum amyloid A (SAA) (16-21).
However, at this time, only hsCRP among the aforementioned markers has been shown to strongly correlate with incident CVD risk and all-cause mortality, including CVD-related mortality, independent of other CVD risk factors, such as non-HDL cholesterol, smoking, or hypertension (14,15,22). This led to its inclusion in the recent 2018-Multisociety guidelines’ recommendation, which advocate testing for hsCRP in patients with intermediate CVD risk, as assessed by conventional CVD risk biomarkers, providing guidance on how aggressively such patients should be treated (23). However, despite its good analytical performance in terms of its long-term stability in frozen blood samples, and its readily available assays in most laboratories (13), hsCRP carries certain limitations as a CVD biomarker, particularly with regards to its high biological variability, where even a mild recent or current infection, or a localized site of inflammation, can remarkably increase its value for several days, or even weeks. Moreover, there is a high degree of intra- and inter-assay variability of hsCRP, which precludes the reliability of trending its levels over time (24,25). Finally, hsCRP levels also appear to increase with age, and are generally higher in women compared with men (26). Thus, given these limitations of hsCRP, there is growing interest in identifying alternative inflammatory biomarkers for CVD risk prediction. Such biomarkers must have a better credibility than hsCRP, specifically, higher precision and accuracy (27).
GlycA: a new marker of systemic inflammation
Since its first report in 2014, the GlycA test has been has been gaining increasing interest, particularly as a new biomarker for CVD risk assessment (28-30). The test itself, and its clinical utility however, remain poorly understood by the majority of clinicians, from primary care physicians to cardiologists. In this short review, we briefly describe what the test measures, how it may be used for CVD risk prediction, and compare it with hsCRP, another inflammatory biomarker that can be used to predict CVD risk (1,14,15,17).
What is GlycA?
In order to understand what the GlycA test measures, a brief understanding of the inflammatory process is essential, which will be presented below. Under states of acute or chronic inflammation, irrespective of the underlying trigger (i.e., infection, trauma, etc.), leukocytes are recruited to the site of inflammation, where they secrete a variety of pro-inflammatory cytokines, mainly interleukin-1 (IL-1), IL-6, and tumor-necrosis factor alpha (TNF-alpha) (31,32). These cytokines have local pro-inflammatory effects, but in the case of IL-6, and IL-1 and TNF-alpha to a lesser degree, they enter circulation to exert more systemic effects, namely induce an increased hepatic production and secretion of several so-called “acute phase reactants”. These primarily include fibrinogen, CRP, haptoglobin, SAA, and many other proteins (31,32). An interesting feature of these acute phase reactants is that they are almost all N-linked glycoproteins, i.e., they all contain oligosaccharide chains attached to the side chain nitrogen group of several of their asparagine residues (31,33). Hence, the overall number of these N-glycan oligosaccharide branches, markedly increases in the setting of acute inflammation, mirroring the increase that occurs in the individual levels of the corresponding acute phase reactant proteins (34,35). However, because the inflammatory process itself also evolves and amplifies over time, these N-glycosylation “patterns” also evolve in their complexity, as the subtypes and activities of the different intracellular and secreted hepatic glycosyltransferases also evolve during the inflammatory cascade. Notable changes affecting these N-glycan composition include further extensions of the glycan residues, increasing the branching of existing ones, or modifying them by removing sialic acid or galactose residues (34-36).
The GlycA test was developed as a composite or overall measure of changes in both, the number and the complexity of N-glycan side chains attached to acute phase reactant proteins (34,35). The GlycA “signal” that is measured, is essentially the proton nuclear magnetic resonance (1H-NMR) spectroscopy signal of the methyl groups found on N-acetylglucosamine residues attached to all circulating plasma proteins (34,36,37). However, because the majority of circulating N-glycosylated proteins are acute phase reactants, the GlycA test thereby reflects changes in both the overall levels, and the oligosaccharide chain complexity of acute phase reactant proteins (Figure 1). It is also worth mentioning here that, five acute phase reactants in particular, contribute the most to the GlycA signal, namely alpha-1-acid glycoprotein (AGP), alpha-1-antitrypsin (AAT), alpha-1-antichymotrypsin (AACT), haptoglobin, and transferrin (34,38), among which, AGP and AAT are particularly important, given their independent correlations with CVD and other disease states (30,38,39).
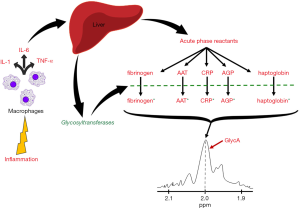
GlycA: a possible alternative to hsCRP for CVD risk assessment
GlycA levels have been shown to positively correlate with both, hsCRP and IL-6 levels, in several studies (29,34,40,41), as well as with fibrinogen levels (34). The correlation coefficient between GlycA and hsCRP levels was only moderate, ranging between 0.45 and 0.67 (28-30,42), which suggests that, while both tests overlap in ‘sensing’ similar aspects of systemic inflammation, they also possibly cover different aspects of the inflammatory process (43,44). In fact, unlike hsCRP, which is a measure of a discrete inflammatory protein, GlycA is a composite biomarker that measures the extent and complexity of N-glycosylation of various plasma proteins, and therefore, the overall inflammatory “status” in the body (34). In a sense, GlycA can be thought of as the inflammatory marker-analogue of hemoglobin A1c (HbA1c), which is a stable biomarker of glucose homeostasis that unlike plasma glucose level, does not exhibit significant day-to-day or post-prandial variations (45). Likewise, compared with hsCRP that has remarkable intra- and inter-assay variability (24,25,46,47), GlycA is more stable, having a remarkably lower biological variability (~5% vs. ~30% for hsCRP) (34,46). Additionally, hsCRP levels on average, are 40% higher in women compared with men, and positively correlate with age and race, according to several studies (42,48,49). In contrast, while some studies have shown that women have higher baseline GlycA levels compared with men, these differences did not exceed 10% however. As such, GlycA levels do not exhibit high variability across the two sexes, like hsCRP does (30,46). Nonetheless, it remains imperative to establish specific “reference” ranges for GlycA levels, for each of the two sexes, especially since sex hormones have been shown in multiple studies to influence the inflammatory process, including the degree and extent of N-glycosylation of proteins (50,51).
Available evidence on GlycA as a CVD biomarker
Observational studies
Several prospective cohort studies on the predictive value of GlycA for incident CVD events, have shown a significant positive correlation between elevated baseline GlycA levels and future CVD events (28-30,47), with these correlations maintaining significance after adjustment for other CVD risk factors (28-30,47). Among the first studies evaluating GlycA levels as a CVD risk biomarker, was the Women’s Health Study (WHS), which is the study with the largest sample size and longest follow up period conducted to date, demonstrating a positive correlation between baseline GlycA levels and future CVD events (N=27,491 participants; median follow up: 17.2 years) (28). It is also the first to show a graded correlation between baseline GlycA levels and incident CVD events, such that the risk of future CVD events gradually increases from individuals within the lowest quartile range of baseline GlycA levels, to those in the highest quartile (28). This graded correlation was subsequently replicated in the Prevention of Renal and Vascular End-Stage Disease (PREVEND) study, which included both men and women (N=4,759 participants; median follow up: 8.5 years) (30). It also included patients with microalbuminuria, demonstrating that the correlation between baseline GlycA levels and future CVD event rates was not only graded, but also independent of renal function (30). Shortly afterwards, the Multi-Ethnic Study of Atherosclerosis (MESA), which had a slightly larger sample size and longer median follow up period (6,523 participants; median follow up: 12.1 years) than PREVEND, generated similar findings with regards to the predictive value of GlycA for incident CVD events (29). This was also the first study to reveal that women have slightly higher baseline GlycA levels compared with men (29).
Interventional studies
During the same year as the MESA study, a post-hoc analysis on a subset of participants included in the Justification for the Use of Statins in Prevention: an Intervention Trial Evaluating Rosuvastatin (JUPITER) trial (N=10,039 participants; median follow up: 1.9 years), also showed a significant correlation of baseline GlycA levels, graded into quartiles, with future CVD event rates (47). These correlations were slightly attenuated after adjustment for hsCRP, but remained statistically significant nonetheless (47). The importance of this trial in particular, was showing that patients with similar GlycA levels who received statin therapy had lower CVD incidence rate, compared with their counterparts who were not receiving statins. The magnitude of reduction in incident CVD event rates in statin versus placebo groups however, was intriguingly similar among the designated GlycA quartiles (47). The authors of that study hypothesize that this somewhat “fixed” effect of statin therapy to reduce incident CVD event rates, across the different GlycA quartile ranges, suggests that statins only target part of the “pathway” associating elevated GlycA levels and CVD risk, with GlycA “covering” other residual pathways involved in the development and progression of atherosclerosis, which if targeted, can augment statin therapy in further reducing CVD risk (47).
GlycA correlation with other CVD biomarkers
Several of the aforementioned studies also went on to assess the correlation between baseline GlycA levels, and several other well-established CVD risk factors, demonstrating a moderately positive degree of correlation, between baseline GlycA levels, and hsCRP, total cholesterol, low-density lipoprotein cholesterol (LDL-C), body mass index (BMI), smoking, hypertension, and triglyceride levels, individually (28-30,34,43,47).
GlycA association with mortality and non-CVD conditions
Recent studies also demonstrate a positive correlation between baseline GlycA levels and all-cause mortality, including CVD-related mortality (29,52). This association was mildly attenuated, but retained significance, after adjusting for several CVD-related biomarkers, such as hsCRP, IL-6, D-dimer, etc. (29,52). This association was subsequently replicated in the retrospective analysis performed on biorepository samples of the Catheterization Genetics (CATHGEN) study (N=7,617 participants), which evaluated GlycA levels in a cohort of patients undergoing cardiac catheterization (53). Specifically, that study was the first to show that GlycA levels significantly associate with both, the presence, and extent of coronary artery disease (53). Overall, the predictive value of GlycA levels for incident CVD events and CVD-related mortality have been shown to be comparable with those of hsCRP (29,30,47). However, while baseline GlycA levels remain significantly associated with all-cause mortality after adjusting for several CVD-related risk factors, hsCRP loses significance after adjusting for the same biomarkers (29,54). Moreover, several studies have shown that baseline GlycA levels positively correlate with incident type 2 diabetes mellitus, in a graded response that has a similar pattern to that of hsCRP (42,46,55). This correlation was slightly attenuated, but remained significant, after adjustment for HbA1c, highlighting a key distinction between the glycation processes measured by either test; GlycA measures enzymatic glycosylation, while HbA1c measures non-enzymatic glycosylation (55). In addition, GlycA levels showed mild inverse correlation with insulin sensitivity (r=−0.33), a correlation similar but weaker than that of hsCRP with insulin sensitivity (r=−0.41) (42). Finally, only baseline GlycA levels, but not hsCRP or IL-6, showed statistically significant correlation with cancer incidence and cancer-related mortality, with this association however, retaining significance only in men and non-white ethnicities, after adjusting for several risk factors (29,54). In contrast, neither GlycA nor hsCRP levels have been shown to significantly associate with stroke incidence (29).
It is worth noting that GlycA levels are not invariant; they change in response to various treatments, primarily with anti-inflammatory agents, as well as in response to behavioral (i.e., lifestyle) modifications. These attributes in particular, grant GlycA the ability to reflect an overall status of systemic inflammation in the body, which offers greater advantage compared with other biomarkers, such as lipid levels. For example, GlycA levels have been shown to decrease with physical activity (56-58), and weight loss, irrespective of whether the latter is achieved through dietary modifications (i.e., caloric restriction) or medical interventions (e.g., bariatric surgery) (57,59,60). Moreover, GlycA levels also decrease in response to treatment with different anti-inflammatory biological therapeutics, such as anti-TNF-alpha antibodies (i.e., infliximab or adalimumab) (61,62), Interleukin-12 and Interleukin-23 inhibitors (i.e., ustekinumab) (63), and Janus kinases 1 and 2 (JAK1/2) inhibitors (i.e., baricitinib) (64,65). On the other hand, GlycA levels failed to show significant reductions in their levels in response to other CVD treatments such as statins (47) or niacin (66), or anti-inflammatory therapies, such as methotrexate and corticosteroids (67).
GlycA: future directions and implications
As with any new diagnostic test, a lot remains unanswered about the GlycA test. For a start, we propose additional studies testing GlycA levels in the general population or select subsets of it, in order to identify the various pathological and/or physiological conditions affecting its levels (68). Additional studies are also needed to help establish the clinical utility of the GlycA test as a CVD risk-predictor biomarker, focusing particularly on understanding its predictive value compared with that of hsCRP, specifically in patients with intermediate CVD risk.
On the other hand, in the event that specific anti-inflammatory therapies targeting CVD become available in the future, GlycA levels could be a useful biomarker for monitoring response to such therapies, given its well-established correlation with inflammation. Thus, for the time being, it would be worthwhile monitoring GlycA levels, as well as hsCRP levels, in any current or prospective clinical trials testing anti-inflammatory therapies for CVD treatment.
A major limitation that would preclude a widespread use of the GlycA test, is its need for an NMR analyzer, which is not available in the majority of routine clinical laboratories. Moreover, the specific GlycA assay with which most GlycA-relevant studies reported to date in the literature have been conducted, is that developed by LabCorp, on the Vantera® NMR clinical analyzer (34,46). However, with increasing interest in GlycA levels, and determining their “trends” in various health conditions, several laboratories have subsequently developed their own GlycA tests that use different NMR analyzers than the Vantera® analyzer. These different assays have also been found to report “higher” GlycA levels compared with those reported by LabCorp on the Vantera® analyzer (43). Such differences raise questions about the degree of comparability among the different results generated from those different NMR analyzers. They also raise concern for whether these different GlycA assays actually measure the same “species” in plasma, and if they do, try to explain why they report multiple-fold differences in their measured levels. Such questions remain key to be answered and clarified, before the GlycA test can become standardized and available in different laboratories.
Nonetheless, it is this complexity of measuring GlycA levels on an NMR analyzer that also paradoxically offers an advantage to the test. In addition to measuring GlycA, the same NMR analyzer can also be used to simultaneously measure total serum lipids, including lipoprotein particle counts and sizes, as well as other CVD-related biomarkers, all within a single run of each sample. This all-in-one approach of analyzing a specimen, may facilitate the identification of different interactions between GlycA levels and several of these ‘other’ NMR-detected species, which would likely drive a further increased utilization of the test, by adding more clinical ‘value’ to it.
In conclusion, GlycA is a relatively recently discovered biomarker of systemic inflammation that is rapidly evolving into a potential biomarker predictor of CVD risk, and a quantitative entity for monitoring response to various anti-inflammatory therapies undergoing investigation for CVD risk attenuation.
Acknowledgments
Funding: This work was supported by the Intramural Research Program of the National Heart, Lung, and Blood Institute (NHLBI) under grant number [HL006092] at the National Institutes of Health (NIH).
Footnote
Provenance and Peer Review: This article was commissioned by the Guest Editors (Sridevi Devaraj and Jing Cao) for the series “Cardiovascular Precision Medicine” published in Journal of Laboratory and Precision Medicine. The article has undergone external peer review.
Conflicts of Interest: Both authors have completed the ICMJE uniform disclosure form (available at http://dx.doi.org/10.21037/jlpm.2020.03.03). The series “Cardiovascular Precision Medicine” was commissioned by the editorial office without any funding or sponsorship. The authors have no conflicts of interest to declare.
Ethical Statement: The authors are accountable for all aspects of the work in ensuring that questions related to the accuracy or integrity of any part of the work are appropriately investigated and resolved.
Open Access Statement: This is an Open Access article distributed in accordance with the Creative Commons Attribution-NonCommercial-NoDerivs 4.0 International License (CC BY-NC-ND 4.0), which permits the non-commercial replication and distribution of the article with the strict proviso that no changes or edits are made and the original work is properly cited (including links to both the formal publication through the relevant DOI and the license). See: https://creativecommons.org/licenses/by-nc-nd/4.0/.
References
- Albert CM, Ma J, Rifai N, et al. Prospective study of C-reactive protein, homocysteine, and plasma lipid levels as predictors of sudden cardiac death. Circulation 2002;105:2595-9. [Crossref] [PubMed]
- Ruparelia N, Chai JT, Fisher EA, et al. Inflammatory processes in cardiovascular disease: a route to targeted therapies. Nat Rev Cardiol 2017;14:314. [Crossref] [PubMed]
- Willerson JT, Ridker PM. Inflammation as a cardiovascular risk factor. Circulation 2004;109:II2-10. [PubMed]
- Libby P. Inflammation in atherosclerosis. Arterioscler Thromb Vasc Biol 2012;32:2045-51. [Crossref] [PubMed]
- Ridker PM, Luscher TF. Anti-inflammatory therapies for cardiovascular disease. Eur Heart J 2014;35:1782-91. [Crossref] [PubMed]
- Scott DW, Vallejo MO, Patel RP. Heterogenic endothelial responses to inflammation: role for differential N-glycosylation and vascular bed of origin. J Am Heart Assoc 2013;2:e000263 [Crossref] [PubMed]
- van den Boogert MAW, Larsen LE, Ali L, et al. N-Glycosylation Defects in Humans Lower Low-Density Lipoprotein Cholesterol Through Increased Low-Density Lipoprotein Receptor Expression. Circulation 2019;140:280-92. [Crossref] [PubMed]
- Ridker PM, Everett BM, Thuren T, et al. Antiinflammatory Therapy with Canakinumab for Atherosclerotic Disease. N Engl J Med 2017;377:1119-31. [Crossref] [PubMed]
- Hemkens LG, Ewald H, Gloy VL, et al. Colchicine for prevention of cardiovascular events. Cochrane Database Syst Rev 2016;CD011047 [PubMed]
- Everett BM, Pradhan AD, Solomon DH, et al. Rationale and design of the Cardiovascular Inflammation Reduction Trial: a test of the inflammatory hypothesis of atherothrombosis. Am Heart J 2013;166:199-207.e15. [Crossref] [PubMed]
- Stoner L, Lucero AA, Palmer BR, et al. Inflammatory biomarkers for predicting cardiovascular disease. Clin Biochem 2013;46:1353-71. [Crossref] [PubMed]
- Welsh P, Grassia G, Botha S, et al. Targeting inflammation to reduce cardiovascular disease risk: a realistic clinical prospect? Br J Pharmacol 2017;174:3898-913. [Crossref] [PubMed]
- Musunuru K, Kral BG, Blumenthal RS, et al. The use of high-sensitivity assays for C-reactive protein in clinical practice. Nat Clin Pract Cardiovasc Med 2008;5:621-35. [Crossref] [PubMed]
- Emerging Risk Factors Collaboration. C-reactive protein concentration and risk of coronary heart disease, stroke, and mortality: an individual participant meta-analysis. Lancet 2010;375:132-40. [Crossref] [PubMed]
- Kuller LH, Tracy RP, Shaten J, et al. Relation of C-reactive protein and coronary heart disease in the MRFIT nested case-control study. Multiple Risk Factor Intervention Trial. Am J Epidemiol 1996;144:537-47. [Crossref] [PubMed]
- Nicholls SJ, Hazen SL. Myeloperoxidase and cardiovascular disease. Arterioscler Thromb Vasc Biol 2005;25:1102-11. [Crossref] [PubMed]
- Ridker PM, Hennekens CH, Buring JE, et al. C-reactive protein and other markers of inflammation in the prediction of cardiovascular disease in women. N Engl J Med 2000;342:836-43. [Crossref] [PubMed]
- Ridker PM, Rifai N, Stampfer MJ, et al. Plasma concentration of interleukin-6 and the risk of future myocardial infarction among apparently healthy men. Circulation 2000;101:1767-72. [Crossref] [PubMed]
- Johnson BD, Kip KE, Marroquin OC, et al. Serum amyloid A as a predictor of coronary artery disease and cardiovascular outcome in women: the National Heart, Lung, and Blood Institute-Sponsored Women's Ischemia Syndrome Evaluation (WISE). Circulation 2004;109:726-32. [Crossref] [PubMed]
- Sofogianni A, Alkagiet S, Tziomalos K. Lipoprotein-associated Phospholipase A2 and Coronary Heart Disease. Curr Pharm Des 2018;24:291-6. [Crossref] [PubMed]
- Stec JJ, Silbershatz H, Tofler GH, et al. Association of fibrinogen with cardiovascular risk factors and cardiovascular disease in the Framingham Offspring Population. Circulation 2000;102:1634-8. [Crossref] [PubMed]
- Ridker PM, Cushman M, Stampfer MJ, et al. Inflammation, aspirin, and the risk of cardiovascular disease in apparently healthy men. N Engl J Med 1997;336:973-9. [Crossref] [PubMed]
- Arnett DK, Blumenthal RS, Albert MA, et al. 2019 ACC/AHA Guideline on the Primary Prevention of Cardiovascular Disease: Executive Summary: A Report of the American College of Cardiology/American Heart Association Task Force on Clinical Practice Guidelines. J Am Coll Cardiol 2019;74:1376-414. [Crossref] [PubMed]
- Bogaty P, Dagenais GR, Joseph L, et al. Time variability of C-reactive protein: implications for clinical risk stratification. PLoS One 2013;8:e60759 [Crossref] [PubMed]
- Bower JK, Lazo M, Juraschek SP, et al. Within-person variability in high-sensitivity C-reactive protein. Arch Intern Med 2012;172:1519-21. [Crossref] [PubMed]
- Hutchinson WL, Koenig W, Frohlich M, et al. Immunoradiometric assay of circulating C-reactive protein: age-related values in the adult general population. Clin Chem 2000;46:934-8. [Crossref] [PubMed]
- Sauer JM. Biomarker Assay Collaborative Evidentiary Considerations Writing Group, Critical Path Institute (C-Path): Critical Path Institute 2019 June 11, 2019.
- Akinkuolie AO, Buring JE, Ridker PM, et al. A novel protein glycan biomarker and future cardiovascular disease events. J Am Heart Assoc 2014;3:e001221 [Crossref] [PubMed]
- Duprez DA, Otvos J, Sanchez OA, et al. Comparison of the Predictive Value of GlycA and Other Biomarkers of Inflammation for Total Death, Incident Cardiovascular Events, Noncardiovascular and Noncancer Inflammatory-Related Events, and Total Cancer Events. Clin Chem 2016;62:1020-31. [Crossref] [PubMed]
- Gruppen EG, Riphagen IJ, Connelly MA, et al. GlycA, a Pro-Inflammatory Glycoprotein Biomarker, and Incident Cardiovascular Disease: Relationship with C-Reactive Protein and Renal Function. PLoS One 2015;10:e0139057 [Crossref] [PubMed]
- Gabay C, Kushner I. Acute-phase proteins and other systemic responses to inflammation. N Engl J Med 1999;340:448-54. [Crossref] [PubMed]
- Gulhar R, Jialal I. Physiology, Acute Phase Reactants. StatPearls. Treasure Island (FL), 2020.
- Jamieson JC, Kaplan HA, Woloski BM, et al. Glycoprotein biosynthesis during the acute-phase response to inflammation. Can J Biochem Cell Biol 1983;61:1041-8. [Crossref] [PubMed]
- Otvos JD, Shalaurova I, Wolak-Dinsmore J, et al. GlycA: A Composite Nuclear Magnetic Resonance Biomarker of Systemic Inflammation. Clin Chem 2015;61:714-23. [Crossref] [PubMed]
- Connelly MA, Gruppen EG, Otvos JD, et al. Inflammatory glycoproteins in cardiometabolic disorders, autoimmune diseases and cancer. Clin Chim Acta 2016;459:177-86. [Crossref] [PubMed]
- Reily C, Stewart TJ, Renfrow MB, et al. Glycosylation in health and disease. Nat Rev Nephrol 2019;15:346-66. [Crossref] [PubMed]
- Turner GA. N-glycosylation of serum proteins in disease and its investigation using lectins. Clin Chim Acta 1992;208:149-71. [Crossref] [PubMed]
- Ritchie SC, Kettunen J, Brozynska M, et al. Elevated serum alpha-1 antitrypsin is a major component of GlycA-associated risk for future morbidity and mortality. PLoS One 2019;14:e0223692 [Crossref] [PubMed]
- Berntsson J, Ostling G, Persson M, et al. Orosomucoid, Carotid Plaque, and Incidence of Stroke. Stroke 2016;47:1858-63. [Crossref] [PubMed]
- Bartlett DB, Connelly MA, AbouAssi H, et al. A novel inflammatory biomarker, GlycA, associates with disease activity in rheumatoid arthritis and cardio-metabolic risk in BMI-matched controls. Arthritis Res Ther 2016;18:86. [Crossref] [PubMed]
- Dungan K, Binkley P, Osei K. GlycA is a Novel Marker of Inflammation Among Non-Critically Ill Hospitalized Patients with Type 2 Diabetes. Inflammation 2015;38:1357-63. [Crossref] [PubMed]
- Lorenzo C, Festa A, Hanley AJ, et al. Novel Protein Glycan-Derived Markers of Systemic Inflammation and C-Reactive Protein in Relation to Glycemia, Insulin Resistance, and Insulin Secretion. Diabetes Care 2017;40:375-82. [Crossref] [PubMed]
- Connelly MA, Otvos JD, Shalaurova I, et al. GlycA, a novel biomarker of systemic inflammation and cardiovascular disease risk. J Transl Med 2017;15:219. [Crossref] [PubMed]
- Ritchie SC, Würtz P, Nath AP, et al. Systems medicine links microbial inflammatory response with glycoprotein-associated mortality risk. bioRxiv 2015; [Crossref]
- Rohlfing C, Wiedmeyer HM, Little R, et al. Biological variation of glycohemoglobin. Clin Chem 2002;48:1116-8. [Crossref] [PubMed]
- Connelly MA, Gruppen EG, Wolak-Dinsmore J, et al. GlycA, a marker of acute phase glycoproteins, and the risk of incident type 2 diabetes mellitus: PREVEND study. Clin Chim Acta 2016;452:10-7. [Crossref] [PubMed]
- Akinkuolie AO, Glynn RJ, Padmanabhan L, et al. Circulating N-Linked Glycoprotein Side-Chain Biomarker, Rosuvastatin Therapy, and Incident Cardiovascular Disease: An Analysis From the JUPITER Trial. J Am Heart Assoc 2016; [Crossref] [PubMed]
- Lakoski SG, Cushman M, Criqui M, et al. Gender and C-reactive protein: data from the Multiethnic Study of Atherosclerosis (MESA) cohort. Am Heart J 2006;152:593-8. [Crossref] [PubMed]
- Wong ND, Pio J, Valencia R, et al. Distribution of C-reactive protein and its relation to risk factors and coronary heart disease risk estimation in the National Health and Nutrition Examination Survey (NHANES) III. Prev Cardiol 2001;4:109-14. [Crossref] [PubMed]
- Ding N, Nie H, Sun X, et al. Human serum N-glycan profiles are age and sex dependent. Age Ageing 2011;40:568-75. [Crossref] [PubMed]
- Stiles AR, Russell DW. SRD5A3: A surprising role in glycosylation. Cell 2010;142:196-8. [Crossref] [PubMed]
- Lawler PR, Akinkuolie A, Buring J, et al. A novel biomarker of circulating glycoproteins and cardiovascular and all-cause mortality among 39,521 initially healthy adults. J Am Coll Cardiol 2015;65:A1358. [Crossref]
- McGarrah RW, Kelly JP, Craig DM, et al. A Novel Protein Glycan-Derived Inflammation Biomarker Independently Predicts Cardiovascular Disease and Modifies the Association of HDL Subclasses with Mortality. Clin Chem 2017;63:288-96. [Crossref] [PubMed]
- Gruppen EG, Kunutsor SK, Kieneker LM, et al. GlycA, a novel pro-inflammatory glycoprotein biomarker is associated with mortality: results from the PREVEND study and meta-analysis. J Intern Med 2019;286:596-609. [Crossref] [PubMed]
- Akinkuolie AO, Pradhan AD, Buring JE, et al. Novel protein glycan side-chain biomarker and risk of incident type 2 diabetes mellitus. Arterioscler Thromb Vasc Biol 2015;35:1544-50. [Crossref] [PubMed]
- Barber JL, Kraus WE, Church TS, et al. Effects of regular endurance exercise on GlycA: Combined analysis of 14 exercise interventions. Atherosclerosis 2018;277:1-6. [Crossref] [PubMed]
- Bartlett DB, Slentz CA, Connelly MA, et al. Association of the Composite Inflammatory Biomarker GlycA, with Exercise-Induced Changes in Body Habitus in Men and Women with Prediabetes. Oxid Med Cell Longev 2017;2017:5608287 [Crossref] [PubMed]
- Tijardovic M, Marijancevic D, Bok D, et al. Intense Physical Exercise Induces an Anti-inflammatory Change in IgG N-Glycosylation Profile. Front Physiol 2019;10:1522. [Crossref] [PubMed]
- Manmadhan A, Lin BX, Zhong J, et al. Elevated GlycA in severe obesity is normalized by bariatric surgery. Diabetes Obes Metab 2019;21:178-82. [Crossref] [PubMed]
- Olson ML, Renteria-Mexia A, Connelly MA, et al. Decreased GlycA after lifestyle intervention among obese, prediabetic adolescent Latinos. J Clin Lipidol 2019;13:186-93. [Crossref] [PubMed]
- Mehta NN, Shin DB, Joshi AA, et al. Effect of 2 Psoriasis Treatments on Vascular Inflammation and Novel Inflammatory Cardiovascular Biomarkers: A Randomized Placebo-Controlled Trial. Circ Cardiovasc Imaging 2018;11:e007394 [Crossref] [PubMed]
- Joshi AA, Lerman JB, Aberra TM, et al. GlycA Is a Novel Biomarker of Inflammation and Subclinical Cardiovascular Disease in Psoriasis. Circ Res 2016;119:1242-53. [Crossref] [PubMed]
- Dierckx T, Verstockt B, Vermeire S, et al. GlycA, a Nuclear Magnetic Resonance Spectroscopy Measure for Protein Glycosylation, is a Viable Biomarker for Disease Activity in IBD. Journal of Crohn's and Colitis 2018;13:389-94. [Crossref] [PubMed]
- Taylor PC, Kremer JM, Emery P, et al. Lipid profile and effect of statin treatment in pooled phase II and phase III baricitinib studies. Ann Rheum Dis 2018;77:988-95. [Crossref] [PubMed]
- Kremer J, Emery P, Connelly MA, et al. Baricitinib Reduces GlycA Levels in Phase 2 and Phase 3 Clinical Trials in Patients with Moderate to Severe Rheumatoid Arthritis Arthritis Rheumatol 2017;69: [abstract].
- Otvos JD, Guyton JR, Connelly MA, et al. Relations of GlycA and lipoprotein particle subspecies with cardiovascular events and mortality: A post hoc analysis of the AIM-HIGH trial. J Clin Lipidol 2018;12:348-55.e2. [Crossref] [PubMed]
- Ormseth MJ, Chung CP, Oeser AM, et al. Utility of a novel inflammatory marker, GlycA, for assessment of rheumatoid arthritis disease activity and coronary atherosclerosis. Arthritis Res Ther 2015;17:117. [Crossref] [PubMed]
- Ritchie SC, Wurtz P, Nath AP, et al. The Biomarker GlycA Is Associated with Chronic Inflammation and Predicts Long-Term Risk of Severe Infection. Cell Syst 2015;1:293-301. [Crossref] [PubMed]
Cite this article as: Ballout RA, Remaley AT. GlycA: a new biomarker for systemic inflammation and cardiovascular disease (CVD) risk assessment. J Lab Precis Med 2020;5:17.