Altered lipid profile in patients with COVID-19 infection
Introduction
Coronaviruses are a large family of viruses which are known to cause a vast array of diseases, ranging from common cold to more serious conditions such as Severe Acute Respiratory Syndrome (SARS) and Middle Eastern Respiratory Syndrome (MERS) (1,2). Since December 2019, several cases of interstitial pneumonia have been reported in the city of Wuhan, a metropolis in the Chinese province of Hubei (3). Some patients rapidly developed acute respiratory stress syndrome (ARDS), acute respiratory failure, and other serious systemic complications. Deep sequencing analysis from lower respiratory tract samples indicated a novel coronavirus, which was initially named 2019 novel coronavirus (2019-nCoV), and then finally called severe acute respiratory syndrome coronavirus 2 (SARS-CoV-2) (4). The epidemic spread quickly in Asia and subsequently in the rest of the world, affecting several millions people and causing thousands of deaths. On 11 March 2020, the World Health Organization (WHO) finally declared COVID-19 as a pandemic disease.
SARS-CoV-2 is an enveloped virus surrounded by a lipid bilayer, with a genome of approximately 30.000 nucleotides, encoding four structural proteins: the spike (S) protein, the envelope (E) protein, the membrane (M) protein and the nucleocapsid (N) protein (5). Among them, the S protein plays the most important roles in viral attachment, fusion and entry. It mediates the viral entry into host cells through binding its receptor-binding domain (RBD) comprised within the S1 subunit to angiotensin-converting enzyme 2 (ACE2) as host receptor, and then by fusing the viral and host membranes through the S2 subunit (6).
Lipids are essential cell components of SARS-CoV2. In particular, they are involved in fusion of the viral membrane to the host cell, viral replication, as well as endocytosis and exocytosis (6). Cholesterol and lipid rafts play an especially fundamental role in the early stage of cell infection (7).
Among clinical laboratory features, the lipid profile of patients with COVID-19 infection has not been thoroughly investigated. Very recently, a preliminary study described lower serum cholesterol levels in patients with COVID-19 compared to those without, thus suggesting that cholesterol may play an essential role not only in viral replication and internalization, but also in immune activation (8). Therefore, this study was aimed to investigate the lipid profile in a group of COVID-19 patients during the acute phase of infection, and after recovery.
Methods
Study design
This retrospective study was conducted between February 2020 and April 2020 at the Azienda Socio Sanitaria Territoriale (ASST), “Alessandro Manzoni” Hospital in Lecco.
A group of patients positive for SARS-CoV2 infection at hospital admission was selected, based on the fact that test results of triglycerides, total cholesterol, high-density lipoprotein (HDL)-cholesterol and low-density lipoprotein (LDL)-cholesterol and blood count cells were available (Group before). The lipid profile and the hematological parameters were analyzed in the same group of patients after clinical management, when two consecutive naso-pharingeal swab yielded negative results for presence of SARS-CoV2 RNA by means of reverse-transcription polymerase chain reaction (RT-PCR), with the same techniques used for the original diagnosis upon hospital admission (Group after).
The laboratory tests results were compared between these two groups, as well as with a group of healthy subjects (Healthy control), matched for age and sex, without dyslipidemia, diabetes, hypertension and cardiovascular disease, selected among the blood donors attending the Transfusion Center of the ASST-Lecco.
Laboratory tests
The presence of SARS-CoV-2 in nasopharyngeal swab was identified with real-time RT-PCR amplification by GeneFinder COVID-19 PLUS RealAmp Kit (OSANG Healthcare Co, Ltd., Korea) on the ELITe InGenius platform (ELITech Group, Puteaux, France), which integrates extraction and amplification. The real-time PCR kit detects simultaneously the presence of three SARS-CoV-2 targets: envelope protein (E), nucleocapsid protein (N) and RNA-dependent RNA polymerase (RdRp) genes. An internal control, based on amplification of human beta-globin gene, allows to confirm the quality of sample material extracted and test performance.
Biochemical parameters were assessed using the automated clinical chemistry analyzer Dimension Vista 1500 (Siemens Healthcare, Erlangen, Federal Republic of Germany), whilst hematological parameters were obtained using a Sysmex XN 9000 hematology analyzers (Sysmex©, Kobe, Japan).
Statistical analysis
Statistical analysis was performed with Analyze-it software, version 3.90.1 (Analyse-it Software Ltd.; Leeds, UK). All data are reported as median, 95% confidence interval (95% CI) and interquartile range (IQR) after verification of abnormal data distribution by Shapiro-Wilk test. Wilcoxon test was used to compare differences between groups.
The correlation among the various biochemical and hematological parameters was based on spearman’s rank order test. All results were considered significant when the P value was <0.05.
Results
The study population consisted of 55 COVID-19 infected patients (30 males and 25 females; mean age, 54±8.7 years) and 55 healthy control subjects (30 males and 25 females; mean age, 52±7.5 years).
Compared to the healthy controls, SARS-CoV-2-positive patients at baseline (Group before) had lower median levels of total cholesterol, HDL-cholesterol and LDL-cholesterol (3.49 vs. 5.25 mmol/L, 0.71 vs. 1.47 mmol/L and 1.86 vs. 3.18 mmol/L, respectively; all P<0.0001) (Table 1). Conversely, triglyceride levels were found to be higher in SARS-CoV-2 infected patients than in the healthy controls (1.77 mmol/L vs. 1.15, P<0.0001) (Table 1). A similar trend was observed for monocyte/HDL-cholesterol ratio, which was also found to be higher in group before than in the healthy controls (0.73 vs. 0.35; P<0.0001) (Table 1).
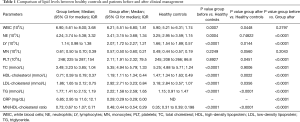
Full table
Comparing healthy controls and group after, statistically significant differences were observed for all parameters except for total cholesterol (Table 1). The triglyceride levels were found to be higher than in the healthy controls also after recovery (Table 1). Notably, no statistically significant differences could be noted for monocytes between group after and the healthy controls, whilst the monocyte/HDL-cholesterol ratio was still higher in group after than in the healthy controls (0.67 vs. 0.35; P<0.0001, Table 1).
In Table 1 also shows the comparison between the lipid profile in SARS-CoV-2-positive patients at the baseline and after recovery. The average time for achieving SARS-CoV-2 negativization was 36±6 days. Total cholesterol, HDL-cholesterol, LDL-cholesterol and triglyceride were found to be significantly higher after recovery than during the acute phase of infection (P<0.0001, Table 1). Moreover, patients in group before had values of monocyte/HDL-cholesterol ratio lower than those found in Group 1 (0.48 vs. 0.73; P<0.001) (Table 1).
The correlation of C-reactive protein, lymphocyte and monocyte with LDL-cholesterol, total cholesterol and HDL-cholesterol in SARS-CoV-2 patients before and after clinical treatment (Group after and before) are shown in Figure 1. C-reactive protein levels were found to be inversely correlated with those of LDL-cholesterol (rs =‒0.573, P<0.0001, Figure 1A), HDL-cholesterol (rs =‒0.700, P<0.0001, Figure 1B) and total cholesterol (rs =‒0.732, P<0.0001, Figure 1C). The lymphocyte count positively correlated with LDL-cholesterol (rs =0.277, P<0.0001, Figure 1D), HDL-cholesterol (rs=0.374, P<0.0001, Figure 1E) and total cholesterol (rs =0.488, P<0.0001, Figure 1F). The monocyte count had no significant association with LDL-cholesterol (rs =‒0.007, P=0.9419, Figure 1G), HDL-cholesterol (rs =0.008, P=0.9375, Figure 1H) and total cholesterol (rs =0.003, P=0.9715, Figure 1I).
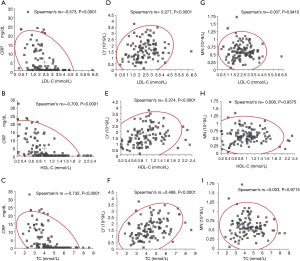
Discussion
This study was basically aimed at evaluating the lipid profile in patients with SARS-CoV-2 during the acute phase of infection and after treatment and recovery, as established by two consecutive negative test results for SARS-CoV2 RNA in naso-pharingeal swabs.
The main finding of this study is that SARS-CoV-2 viral infection and the resulting pro-inflammatory state may have profound impact on lipid metabolism. The major role played by inflammation in determining most lipid abnormalities (i.e., reduced levels of LDL-cholesterol, total cholesterol and HDL-cholesterol) is reflected by the correlation with C-reactive protein and lymphocyte count, as shown in Figure 1. In particular, we found that the initial lipid levels, especially those of total cholesterol, HDL-cholesterol and LDL-cholesterol in patients with SARS-CoV-2 infection were significantly lower than in a healthy control population (P<0.001), whilst triglyceride levels we found to be higher (Table 1). Similar alterations values have been previously emphasized in patients with human immunodeficiency virus (HIV) infection (9,10).
More specifically, Grunfeld et al. showed that HIV patients exhibited increased plasma triglyceride and free fatty acid values, whilst those HDL-cholesterol and total cholesterol were found to be decreased (11). Additional studies showed that HIV-infected patients tend to developed some forms of dyslipidemia, i.e. low LDL-cholesterol levels, hypocholesterolemia and hypertriglyceridemia (12).
Wu et al., in 2017 assayed lipid metabolism in patients recovered from SARS, 12 years after the infection, reporting that triglyceride and very low-density lipoprotein (VLDL) cholesterol values were significantly higher than in healthy controls (13). Similarly, Hu et al. recently reported that total cholesterol, HDL-cholesterol and LDL-cholesterol levels in patients with COVID-19 were lower than in those of a healthy control population (8).The results of these studies are in keeping with those obtained in our investigation, except for triglycerides, which were found to be higher than in the control group in our investigation, both at baseline and after recovery (8).
The HDLs contain triglycerides, free or esterified cholesterol, apolipoprotein (Apo-A-I, Apo-A-II), phospholipids, enzymes and transfer proteins. This class of lipoproteins plays an important role in vasodilatation and in reducing LDL oxidation, thrombosis, apoptosis, inflammation and infection. Besides being an anti-inflammatory lipoprotein with protective effects against lipid oxidation, HDLs negatively regulate T-cells activation and expression of inflammatory mediators in macrophages and dendritic cells (14-17). In serum of patients with COVID-19, the sharply elevated values of pro-inflammatory cytokines indicate the presence of systemic inflammation. HDLs inhibit the expression of adhesion molecules by the endothelium in response to inflammatory cytokines and inhibit the adhesion of monocytes to the endothelium. They also inhibit the activation of monocytes, reduce their secretion of pro-inflammatory cytokines, inhibit activation and diapedesis of neutrophils (18,19). These direct anti-inflammatory properties are complemented and an enhancement of antioxidant functions, which would allow removal of oxidized lipids and neutralization of some oxidative mediators, thus further mitigating the local inflammatory response. deGoma et al. showed that the incubation of endothelial cells with TNFα is effective to stimulate the expression of adhesion molecules such as ICAM-1, VCAM-1, E-selectin and P-selectin, which mediates adhesion and diapedesis of monocytes and other leukocytes within the arterial wall (18). By adding HDL, a significantly reduced expression of these molecules has been noted, thus attenuating infiltration of monocytes within the arterial wall. This effect was shown to be mostly dose-dependent, with maximum inhibiting capacity at physiological HDL concentrations (18,20). Huang et al. reported that the cytokine storm was associated with disease severity (4). During systemic inflammation, HDL can be oxidized (21,22). Oxidized HDL and oxidized LDL were shown to upregulate immune activation (23,24). Data garnered in our study shows that HDL levels in SARS-CoV-2-positive patients were significantly lower during active infection than in healthy subjects. Based on the immunomodulatory effects of HDL-cholesterol, we can hypothesize that these decreased levels were due to the involvement of these lipoproteins in regulation of immune cells during COVID-19 infection. However, further studies would be needed to verify whether HDL and LDL are oxidized in this pathology, and if they negatively regulates activation of T cells and expression of inflammatory mediators in macrophages and dendritic cells.
Most conclusions on the interplay between SARS-CoV-2 infection and lipids are based on previous research, carried out on patients with other human coronaviruses infections. For example, it has been reported that lipid rafts may play a fundamental role in coronavirus life cycle (6,7,25). Lipid rafts are microdomains of eukaryote membrane that contain glycosphingolipids, high concentrations of cholesterol, protein of transport and adhesion. The viral infection depends on interactions between components of plasma membrane of host cell and virus envelope. The presence of cholesterol at the surface of target cells is hence essential for enabling coronavirus infections. In the early stage of SARS-CoV infection, for example, cholesterol and lipid membrane rafts may be essential determinants of viral entry into the cell, as shown for other viral infections (26), whereby viruses attacks these surface molecules on the host cell in specific areas of the plasma membrane characterized by lipid rafts (27). Some cholesterol-rich microdomains facilitate interaction between the spike glicoprotein on SARS-CoV-2 and ACE2, which is localized within the lipid raft (28). In our study, cholesterol values were found to be low during active SARS-CoV-2 infection, while a significant increase was noted after treatment and recovery. This would lead us to hypothesize that the initial decline of cholesterol levels may be associated with SARS-CoV-2 infection, though it is still unclear whether these changes would be somehow associated with viral fusion with host cells and subsequent internalization.
In conclusion, the results of our study seemingly attest that lipids, especially cholesterol, may play an important role in viral replication, internalization and immune activation in patients with SARS-CoV-2 infection. We also speculate that the lipid abnormalities observed during and after this infection could be used for assessing indirectly the response to clinical treatment.
Acknowledgments
Funding: None.
Footnote
Data Sharing Statement: Available at http://dx.doi.org/10.21037/jlpm-20-98
Conflicts of Interest: All authors have completed the ICMJE uniform disclosure form (available at http://dx.doi.org/10.21037/jlpm-20-98). Dr. Giuseppe Lippi serves as an unpaid editor-in-chief of Journal of Laboratory and Precision Medicine. The other authors have no conflicts of interest to declare.
Ethical Statement: The authors are accountable for all aspects of the work in ensuring that questions related to the accuracy or integrity of any part of the work are appropriately investigated and resolved. The entire study was performed in accordance with the Declaration of Helsinki (as revised in 2013) and under the terms of all relevant local legislations. The data obtained from our study did not invalidate the patients clinic. Given the retrospective data analysis nature, informed consent is not required according to our local ethical review board. Test results age and sex of patients were anonymously extracted from the database of the laboratory information system (LIS). For this type study neither informed consent nor ethical committee approval were necessary.
Open Access Statement: This is an Open Access article distributed in accordance with the Creative Commons Attribution-NonCommercial-NoDerivs 4.0 International License (CC BY-NC-ND 4.0), which permits the non-commercial replication and distribution of the article with the strict proviso that no changes or edits are made and the original work is properly cited (including links to both the formal publication through the relevant DOI and the license). See: https://creativecommons.org/licenses/by-nc-nd/4.0/.
References
- Ksiazek TG, Erdman D, Goldsmith CS, et al. A novel coronavirus associated with severe acute respiratory syndrome. N Engl J Med 2003;348:1953-66. [Crossref] [PubMed]
- de Groot RJ, Baker SC, Baric RS, et al. Middle East respiratory syndrome coronavirus (MERS CoV): announcement of the Coronavirus Study Group. J Virol 2013;87:7790-2. [Crossref] [PubMed]
- Lu H, Stratton CW, Tang YW. Outbreak of pneumonia of unknown etiology in Wuhan, China: The mystery and the miracle. J Med Virol 2020;92:401-2. [Crossref] [PubMed]
- Huang C, Wang Y, Li X, et al. Clinical features of patients infected with 2019 novel coronavirus in Wuhan, China. Lancet 2020;395:497-506. [Crossref] [PubMed]
- Zeng W, Liu G, Ma H, et al. Biochemical characterization of SARS-CoV-2 nucleocapsid protein. Biochem Biophys Res Commun 2020;527:618-23. [Crossref] [PubMed]
- Li GM, Li YG, Yamate M, et al. Lipid rafts play an important role in the early stageof severe acute respiratory syndrome-coronavirus life cycle. Microbes Infect 2007;9:96-102. [Crossref] [PubMed]
- Baglivo M, Baronio M, Natalini G, et al. Natural small molecules as inhibitors of coronavirus lipid-dependent attachment to host cells: a possible strategy for reducing SARS-COV-2 infectivity?. Acta Biomed 2020;91:161-4. [PubMed]
- Hu X, Chen D, Wu L, et al. Low Serum Cholesterol Level Among Patients with COVID-19 Infection in Wenzhou, China. Clin Chim Acta 2020;510:105-11. [Crossref] [PubMed]
- Riddler SA, Smit E, Cole SR, et al. Impact of HIV infection and HAART on serum lipids in men. JAMA 2003;289:2978-82. [Crossref] [PubMed]
- Shor-Posner G, Basit A, Lu Y, et al. Hypocholesterolemia is associated with immune dysfunction in early human immunodeficiency virus-1 infection. Am J Med. 1993;94:515-9. [Crossref] [PubMed]
- Grunfeld C, Pang M, Doerrler W, Shigenaga JK, et al. Lipids, lipoproteins, triglyceride clearance, and cytokines in human immunodeficiency virus infection and the acquired immunodeficiency syndrome. J Clin Endocrinol Metab 1992;74:1045-52. [PubMed]
- Funderburg NT, Mehta NN. Lipid Abnormalities and Inflammation in HIV Inflection. Curr HIV/AIDS Rep 2016;13:218-25. [Crossref] [PubMed]
- Wu Q, Zhou L, Sun X, et al. Altered Lipid Metabolism in Recovered SARS Patients Twelve Years after Infection. Sci Rep 2017;7:9110. [Crossref] [PubMed]
- Murphy AJ, Woollard KJ, Suhartoyo A, et al. Neutrophil activation is attenuated by high-density lipoprotein and apolipoprotein A-I in in vitro and in vivo models of inflammation. Arterioscler Thromb Vasc Biol 2011;31:1333-41. [Crossref] [PubMed]
- Taborda NA, Blanquiceth Y, Urcuqui-Inchima S, et al. High-Density Lipoproteins Decrease Proinflammatory Activity and Modulate the Innate Immune Response. J Interferon Cytokine Res 2019;39:760-70. [Crossref] [PubMed]
- Thacker SG, Zarzour A, Chen Y, et al. High-density lipoprotein reduces inflammation from cholesterol crystals by inhibiting inflammasome activation. Immunology 2016;149:306-19. [Crossref] [PubMed]
- Kaji H. High-density lipoproteins and the immune system. J Lipids 2013;2013:684903. [Crossref] [PubMed]
- deGoma EM, deGoma RL, Rader DJ. Beyond high-density lipoprotein cholesterol levels evaluating high-density lipoprotein function as influenced by novel therapeutic approaches. J Am Coll Cardiol 2008;51:2199-211. [Crossref] [PubMed]
- Baker PW, Rye KA, Gamble JR, et al. Phospholipid composition of reconstituted high density lipoproteins influences their ability to inhibit endothelial cell adhesion molecule expression. J Lipid Res 2000;41:1261-7. [Crossref] [PubMed]
- Calabresi L, Gomaraschi M, Franceschini G. Endothelial protection by high-density lipoproteins: from bench to bedside. Arterioscler Thromb Vasc Biol 2003;23:1724-31. [Crossref] [PubMed]
- Kelesidis T, Currier JS, Huynh D, et al. A biochemical fluorometric method for assessing the oxidative properties of HDL. J Lipid Res 2011;52:2341-51. [Crossref] [PubMed]
- Navab M, Reddy ST, Van Lenten BJ, et al. HDL and cardiovascular disease: atherogenic and atheroprotective mechanisms. Nat Rev Cardiol 2011;8:222-32. [Crossref] [PubMed]
- Kelesidis T, Jackson N, McComsey GA, et al. Oxidized lipoproteins are associated with markers of inflammation and immune activation in HIV-1 infection. AIDS 2016;30:2625-33. [Crossref] [PubMed]
- Zidar DA, Juchnowski S, Ferrari B, et al. Oxidized LDL Levels Are Increased in HIV Infection and May Drive Monocyte Activation. J Acquir Immune Defic Syndr 2015;69:154-60. [Crossref] [PubMed]
- Lu Y, Liu DX, Tam JP. Lipid rafts are involved in SARS-CoV entry into Vero E6 cells. Biochem Biophys Res Commun 2008;369:344-9. [Crossref] [PubMed]
- Tang Q, Liu P, Chen M, Qin Y. Virion-Associated Cholesterol Regulates the Infection of Human Parainfluenza Virus Type 3. Viruses 2019;11:438. [Crossref] [PubMed]
- Heaton NS, Randall G. Multifaceted roles for lipids in viral infection. Trends Microbiol 2011;19:368-75. [Crossref] [PubMed]
- Glende J, Schwegmann-wessels C, Al-falah M, et al. Importance of cholesterol-rich membrane microdomains in the interaction of the S protein of SARS-coronavirus with the cellular receptor angiotensin-converting enzyme 2. Virology 2008;381:215-21. [Crossref] [PubMed]
Cite this article as: Roccaforte V, Daves M, Lippi G, Spreafico M, Bonato C. Altered lipid profile in patients with COVID-19 infection. J Lab Precis Med 2021;6:2.