Investigative algorithms for disorders affecting alkalosis: a narrative review
Introduction
The acid base balance in the blood is tightly controlled using the respiratory and renal systems. Acute and chronic compensatory mechanisms facilitate homeostasis, where the respiratory system is used in acute alkalosis, and the renal system is used in chronic alkalosis. Due to the complex interplay of various systems, including compensatory mechanisms, multi-system diseases or concomitant medications, the cause of an alkalotic state may not be immediately apparent (1). As the members of the healthcare team expand, laboratory diagnostic tests are ordered more frequently by people with less experience in either test selection or diagnosis of metabolic disease. Performing the incorrect test can create spuriously abnormal results leading to patient anxiety and further inappropriate tests. This can delay the correct diagnosis, making the process clinically and economically inefficient. Choosing the correct test first time can greatly improve the experience for the patient as well as the quality and efficiency of healthcare. Therefore the following article will focus on the laboratory tests to aid diagnosis of alkalosis, both respiratory and metabolic, to identify the cause of the imbalance. It should be noted that symptoms caused by changes in pH are not well established as they rarely occur in isolation, e.g., are associated with changes in partial pressure of carbon dioxide (pCO2). This article is not meant to replace the many excellent guidelines and reviews on pathophysiology and treatment. We present the following article in accordance with the Narrative Review reporting checklist (available at https://jlpm.amegroups.com/article/view/10.21037/jlpm-22-8/rc).
Methods
The narrative literature review was created by searching Medline, Google Scholar, OMIM and seminal texts. The diagnostic algorithms were then created using the information gathered for the literature review. The literature was searched over the period September 2021 to January 2021. The language was restricted to English. For further information please see supplementary information (Table S1).
Biological mechanism of alkalosis
The metabolic conversion of carbon dioxide to hydrogen and bicarbonate ions, metabolised by carbonic anhydrase, is the basic chemical step responsible for the acid-base state of the circulating plasma (see below) (2).
Alkali in diet varies widely; however, diets with a lower meat content (vegetarian or vegan) are more likely to be alkaline. Bicarbonate is reabsorbed by the kidneys and carbon dioxide excretion is regulated to expel excess acid production (Figures 1,2) (3).
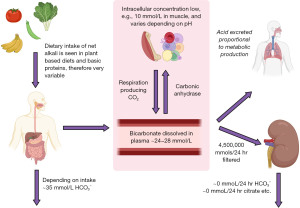
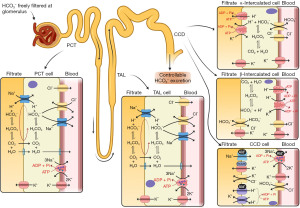
Compensation
The pCO2 is imperative to determine the cause of an acid-base imbalance (Figure 3) and, depending on how acute the problem is, you may see signs of compensation developing (1). Acute compensation involves retaining acid in the form of carbon dioxide by reducing ventilation. The kidneys normally reabsorb 100% of all bicarbonate (Figures 1,2) (4). In chronic alkalosis, the kidneys reduce bicarbonate reabsorption, and this becomes more pronounced as the state progresses in severity. These changes in pCO2 and bicarbonate concentration can be estimated (particularly if the time of onset and type of alkalosis is known) (Table 1) (1). It is important to note that over-compensation does not occur and the compensation formulae were originally established in canine models (5). Most of these have been replaced by formulae derived from small human studies but although linear, there is scatter, and therefore these formulae are estimates and are affected by time of onset, presence of mixed disorders and treatments.
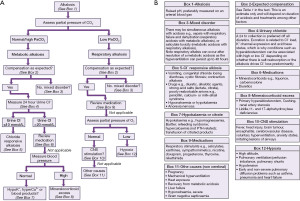
Table 1
Type of alkalosis | Change |
---|---|
Fall in [HCO3−] per decrease in PaCO2 | |
Acute respiratory alkalosis | 0.2 mmol/L per mmHg (0.133 kPa) |
Chronic respiratory alkalosis | 0.4 mmol/L per mmHg (0.133 kPa) |
Rise in PaCO2 per increase in [HCO3−] | |
Metabolic alkalosis | 0.7 mmHg (0.09 kPa) per mmol/L |
[K+]p change for every 0.1 elevation in pH | |
Respiratory alkalosis | 0.3 mmol/L reduction |
Metabolic alkalosis | 0.2 mmol/L reduction |
Acute meaning within hours and chronic several days. PaCO2, arterial partial pressure of carbon dioxide; p, plasma.
Consequences of alkalosis
Hyperchloraemic hypokalaemia
Alkalosis largely affects potassium, causing prominent hypokalaemia (Table 1) (4). This is due to H+ exchange with intracellular K+, and increased K+ excretion via renal sodium pump and apical tubular potassium permeability activation (in an attempt to retain H+). Renal compensation, i.e., bicarbonate excretion, requires anion exchange in the kidneys and tissues in order to maintain electrical neutrality. Bicarbonate is exchanged with chloride ions, which is why alkalosis is associated with hyperchloraemic hypokalaemia (4).
Hypophosphataemia
Hypophosphataemia is seen in alkalosis, as the change in cellular pH stimulates phosphofructokinase, which increases glycolysis and ATP production (6). Extracellular phosphate therefore shifts intracellularly to satisfy the demand.
Hypocalcaemia and hypomagnesaemia
Other laboratory parameters affected by alkalosis include a decrease in ionised (not total) serum calcium, as the positive binding sites on proteins, mainly albumin, are no longer occupied with protons (7). The same process affects magnesium although to a lesser degree, as a lower concentration of magnesium binds albumin (30% for magnesium, rather than 50% for ionised calcium) (8). The person may manifest symptoms of hypocalcaemia and hypomagnesaemia due to low ionised concentrations. However, there may be no measurable abnormality as the total serum concentrations may not change, and ionised calcium is adjusted for pH in most blood gas reports.
Hyperlactataemia
Hyperlactataemia can be caused by alkalosis, primarily due to the stimulation of glycolysis. The increased pyruvate production drives lactate production, and the alkalosis stimulates bicarbonate loss in the kidneys, which in turn prevents renal lactate excretion (to maintain electroneutrality as lactate is negatively charged) (7). Alkalosis also causes a shift in the oxygen dissociation curve, reducing oxygen delivery to tissues and increasing anaerobic respiration (and lactate concentration). The reduction in oxygen delivery causes hyperventilation (which blows off more carbon dioxide exacerbating alkalosis), and the alkalosis also impairs mitochondrial respiration (producing more lactate) (9). Lactate also causes vasoconstriction, exacerbating tissue hypoxia and hyperlactataemia (10). This all can complicate the picture and make diagnosis difficult in some rare cases.
Pre-analytical factors: patients, specimens and laboratory tests
Patient preparation
Pre-analytical issues must be considered when assessing acid-base disorders, and although rarer, analytical issues can also occur. The patient must be breathing at their basal rate when the sample is taken, and they should be prone and as relaxed as possible (10,11).
Specimen tubes and collection vessels
Specimen tubes should be glass, but are mostly plastic, which is more practical, but slightly permeable to oxygen. All air should be expelled from syringes first and only heparin should be used as an anticoagulant, as citrate, EDTA and fluoride alter blood pH (12). Underfilling specimen tubes, which contain liquid anticoagulant, can lead to dilution artefact (reducing pCO2 and bicarbonate concentration), therefore liquid containing tubes should be filled to their maximum (12). Heparin can be acidic, therefore manufacturing variation may result in spurious acidosis (13). Specimens should be analysed immediately, if possible, to prevent metabolism in vitro affecting results (12).
Specimen type
If venous blood is measured instead of arterial, there will be differences in the respiratory gases (high pCO2) (14). The issue is that the expected differences may apply to well, stable, patients; and the differences may be considerably more marked in severely ill patients (4). However, adjustment formulae have been validated in an attempt to limit arterial venesection, which is not without risk, and performance may be acceptable as long as the limitations are understood (15). Capillary sampling is also not recommended, as is affected by changes to the peripheral circulation (12). However, using sites such as the ear lobe that have been ‘arterialised’ (warmed and rubbed to create hyperaemia) first can improve the utility. This is not an appropriate method for pO2, and both pH and pCO2 can be affected by sampling technique (12). In the following paper, we will refer to measurements performed on arterial samples for blood gases (or serum for other analytes).
Patient temperature
Blood gas analysers measure pH, pCO2, and pO2; and then calculate the rest of the derivate parameters. These values are then also adjusted for patient temperature. This practice, albeit controversial, will affect results, depending on whether users correctly enter temperature upon analysis (12). It is true, however, that the ‘reference intervals’ apply; irrespective of the temperature of the patient. The alveolar-arterial gradient must use temperature adjusted values to avoid spurious results (16).
Diagnostic algorithms
An algorithm providing suggestions on an approach to the diagnosis of alkalosis is provided (Figure 3), followed by a high bicarbonate in isolation, for example, in cases where you do not have an arterial blood gas analysis (Figure 4 and see article in same series on acidosis). If the acid-base status is not known, then a high bicarbonate is effectively either a metabolic alkalosis, or compensation for respiratory acidosis. Finally an algorithm specifically looking at hypokalaemic metabolic alkalosis is provided (Figure 5). Importantly, the tests for an acid-base disorder are never completely diagnostic, and the conclusion must be weighed by the clinical evidence (2). Pre-existing acid base disorders, mixed disorders, renal impairment, fluid status, and other therapies, will all affect the results; so correlate closely with the clinical situation (2).
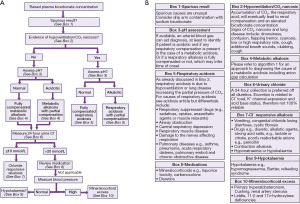
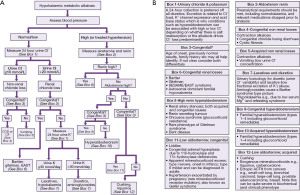
Cautions with urine testing
Urinary measurement is recommended, provided that renal function is normal, and the patient is off all diuretics for as long as possible, e.g., 2 weeks (17). Note for chloride that concentrations between 10 and 20 mmol/L are missing (18). There is variation in the literature such as a single threshold of 20 or 15 mmol/L (19). However, due to the multiple factors affecting urinary excretion, intermediate results may not be diagnostic.
Fractional excretion of sodium is not recommended for distinguishing the cause of hypokalaemic metabolic alkalosis; demonstrating another limitation of this type of measurement (20). Basically, at a steady state, you cannot excrete significantly more or less electrolyte than you intake, therefore, eventually, irrespective of the underlying pathophysiology, the body adapts to keep the ‘high’, or ‘low’, electrolyte state steady (20).
Cautions with aldosterone and renin sampling
Aldosterone and renin are important tests in the algorithms. Effectively, fluid status and salt intake should be adequate, and potassium should be normal before sampling for these tests. If salt intake is increased prior to the test, it can worsen the hypokalaemia. Antihypertensive drugs and patient posture will also affect the results and chilling the sample for renin is problematic (for example, user wrongly transported the sample on ice) (21). Consider stopping diuretics four weeks prior to the test, and β-adrenergic blockers, α-2 agonists (e.g., clonidine, α-methyldopa), non-steroidal anti-inflammatory drugs, angiotensin-converting enzyme inhibitors, angiotensin receptor blockers, renin inhibitors, and dihydropyridine calcium channel antagonists, perhaps more controversially, 2 weeks before the test (7). It is worth noting that the ratio can be less affected by preanalytical factors, such as drugs, than the isolated measurements.
Alkalosis
Respiratory alkalosis
Respiratory alkalosis is caused by hyperventilation, which blows off carbon dioxide (a respiratory acid), hence patients are diagnosed with respiratory alkalosis if they present with an elevated blood pH and low pCO2 (7). Bicarbonate should be low however the reduction is dependent of the time of onset and bicarbonate may be normal in acute respiratory alkalosis (1). Common causes of pure respiratory alkalosis include anxiety attacks, in which the patient hyperventilates, and asthma attacks, where the patient hyperventilates to maintain pO2 but consequently blows off carbon dioxide (7). A normal pCO2 identified during an asthma attack is a poor prognostic indicator demonstrating respiratory fatigue (22).
Metabolic alkalosis
Metabolic alkalosis is typically caused by either an increase in other alkaline agents or renal/gut loss of protons. Arterial blood gasses show elevated pH, bicarbonate and pCO2 (1). Other laboratory findings include: decreased serum ionised calcium, hypokalaemia and hypophosphataemia (see above).
Chloride concentration can be useful due to the effect of aldosterone (see article in series on chloride).
Metabolic alkalosis: saline responsive
Aldosterone is secreted primarily in response to hypovolaemia, and stimulates salt (NaCl) and water reabsorption in the kidney. To maintain electroneutrality, sodium is exchanged for potassium and hydrogen ions. This results in an alkalosis that should respond to saline treatment (called ‘saline responsive’ alkalosis) and is indicated by a spot urine chloride concentration ≤10 mmol/L (or 20 mmol/L, see above) (12). Causes include: losses from the gut (vomiting, suction, diarrhoea), or skin [cystic fibrosis (CF)], and drugs (exogenous alkali, previous diuretic therapy) (18). Chloride responsive alkalosis can also be seen in anorexia nervosa and calcium-alkali (milk-alkali) syndrome (23).
Metabolic alkalosis: calcium-alkali syndrome
Calcium-alkali syndrome is defined by (hypokalaemic) metabolic alkalosis, hypercalcaemia, and renal failure; it occurs following ingestion of compounds such as calcium salts, dairy products, magnesium oxide and sodium bicarbonate for osteoporosis or gastric ulcer treatment (24). Note that hypercalcaemia from malignant causes can cause a saline unresponsive metabolic alkalosis due to release of buffers (e.g., phosphate) from bone (25).
Other causes of metabolic alkalosis
Other causes of volume and/or electrolyte losses (and secondary hyperaldosteronism) include drug abuse or surreptitious vomiting. Laxative abuse can be identified by direct measurement of drugs and metabolites in urine, however a low fractional excretion of potassium can be suggestive (26). Furthermore, urine toxicology can detect diuretic abuse, although urine chloride variability is suggestive (27). Use of thiazide diuretics resembles Gitelman syndrome, with hypochloraemia, hypokalaemia and hypomagnesaemia, with inappropriately high urine chloride if taking medication (see below and Figure 5) (27). Loop diuretics will also cause a hypochloremic, hypokalaemic metabolic alkalosis, but may also demonstrate hyperuricaemia and hypercalciuria (like Bartter syndrome) (28). In suspected bulimia/surreptitious vomiting, low urine chloride concentration can be suggestive (29).
Metabolic alkalosis: saline unresponsive
If urine chloride is ≥20 mmol/L, this suggests it will be saline unresponsive or there is massive renal chloride loss in diuresis (18). Refeeding syndrome causes a saline unresponsive metabolic alkalosis by raising bicarbonate levels and possibly proton movement to the intracellular compartment (30). Blood transfusion containing citrate is also a cause, due to citrate being metabolised to bicarbonate (31).
Primary hyperaldosteronism is indicated by the combination of hypertension, low serum renin, and raised plasma aldosterone:renin activity ratio (32). There are a number of rare causes of metabolic alkalosis, which can mimic hyperaldosteronism among others (32). Blood pressure and aldosterone concentration can aid diagnosis (32).
Mixed disorders of alkalosis
The presence of mixed disorders can complicate diagnosis. One example is salicylate toxicity, as it is a respiratory stimulant, but salicylate in higher concentrations can also cause vomiting and hence a metabolic alkalosis (33). Additionally, salicylate is an acid itself. Use of diuretics (metabolic alkalosis) with chronic lung disease (respiratory acidosis), and vomiting (metabolic alkalosis) with renal failure (metabolic acidosis), are some other examples of mixed disorders (34). The combination of respiratory and metabolic alkalosis can also exacerbate, and complicate, the diagnosis; this is seen in hyperventilated patients with gastric losses.
Pregnancy and paediatric values
Pregnancy
Pregnancy leads to haemodilution, changes in the respiratory system, and reduction in bicarbonate concentration by 2–6 mmol/L (25%), effectively causing a fully compensated respiratory alkalosis (35,36). However, it is important not to disregard a low bicarbonate concentration automatically during pregnancy, particularly if they are presenting with possible metabolic acidosis. Breathless pregnant women have lost some of their buffering capacity and so are more sensitive to acidosis. Blood pH may increase slightly in pregnancy; arterial blood by 0.01 at both the upper and lower end of the normal range, and slightly higher in venous blood (9).
Rare pregnancy causes of alkalosis
It is worth noting that there is a very rare mutation in NR3C2 gene for the mineralocorticoid receptor, which makes it sensitive to other steroids such as progesterone. A syndrome like Liddle syndrome, called ‘hypertension exacerbated by pregnancy’ or Geller syndrome, is observed in these patients (low renin activity, low aldosterone, hypokalaemia, hypertension with metabolic alkalosis) (37). This is distinct from other causes of hypertension, like pre-eclampsia, and may present as hypokalaemia during pregnancy also (38,39).
Paediatric considerations
Children approach adult pCO2 by 5 years of age, but neonates have a lower pCO2 that increases over time (40). The pH and bicarbonate concentrations are assumed to be equivalent to adult normal reference intervals.
Rare conditions and complicating issues
There are some rare causes of hypokalaemic metabolic alkalosis (Figure 5). These conditions will be discussed below, starting with those associated with hypertension.
Rare causes associated with hypertension
Apparent mineralocorticoid excess (AME)
AME syndrome causes hypertension and a hypokalaemic metabolic alkalosis. AME patients have inactivating mutation in the HSD11B2 gene encoding 11β-hydroxysteroid dehydrogenase type 2, which converts cortisol to a form that is inactive at the mineralocorticoid receptor, cortisone (32). This is identical to the effect of liquorice, where glycyrrhizic acid, or glycyrrhetinic acid, inhibit the same enzyme. This enzyme is also saturated in hypercortisolaemia, and hence why Cushing and the rarer cause, ectopic ACTH secreting tumours, also cause metabolic alkalosis with hypokalaemia; due to cortisol activation of the mineralocorticoid receptor (32).
There are two types of AME; type I, a more severe form, presenting in infancy with failure to thrive and prominent hypertension, and a much milder type II, which is usually diagnosed in adulthood (41). Depending on local availability of tests to confirm AME, urine steroid profiles help isolate the enzyme deficiency, as well as genetic testing (42). However, blood pressure, and renin activity and aldosterone measurements, are critical for preventing misdiagnosis of Bartter syndrome (6).
Liddle syndrome
Liddle syndrome is caused by activating mutations in the subunits genes SCNN1A, SCNN1B, SCNN1G of the epithelial sodium channel (ENaC) that is usually regulated by aldosterone (37). Again, Liddle syndrome is another cause of hypertension with hypokalaemic metabolic alkalosis (37). Although onset occurs in childhood, it has been picked up in adults as the condition, although autosomal dominant, has wide phenotypic variation, with some being normokalemic and normotensive (11). Phenotypically, it resembles primary hyperaldosteronism, but has low renin activity and aldosterone concentration, and urine steroids missing the characteristic abnormalities found in AME and congenital adrenal hyperplasia (CAH) (37). Genetic confirmation is needed for a final diagnosis (37).
Geller and Chrousos syndrome
Geller syndrome is another rare syndrome that mimics hyperaldosteronism and Liddle syndrome, due to an activating mutation of the mineralocorticoid receptor (see pregnancy section). In addition, mutations in the glucocorticoid receptor make it insensitive, and results in increased secretion of steroid hormones, inducing hyperaldosteronism (43). Therefore, Chrousos syndrome, or primary generalised familial, or sporadic, glucocorticoid resistance is another cause, however in this case the aldosterone concentration will be high (15).
CAH
Aldosterone synthesis defects occur in patients with CAH. However, metabolic alkalosis will not normally occur unless 11-deoxycorticosterone concentration is increased, leading to enhanced mineralocorticoid activity (33). Therefore, the enzyme deficiencies of 11β-hydroxylase and 17α-hydroxylase can cause hypertension, and hypokalaemic metabolic alkalosis, unlike the usual phenotype associated with CAH (44).
Familial hyperaldosteronism (FH)
FH is a collection of autosomal dominant genetic disorders causing an over production of aldosterone (45-47). FH Type 1 (FH-1) is known as glucocorticoid-remediable aldosteronism (GRA), an autosomal dominant condition where the genes CYP11B1 and CYP11B2 (aldosterone synthase) are fused, causing aldosterone secretion to become regulated by adrenocorticotrophic hormone (ACTH). Aldosterone is switched off in the presence of glucocorticoids, such as dexamethasone, which suppress ACTH. Clinically, it presents as moderate to severe hypertension that is fairly resistant to treatment, usually presenting at under 30 years of age. There is phenotypic variation in FH-1, so family history of primary aldosteronism may not be immediately obvious, but there may be a history of premature haemorrhagic stroke e.g., <40 years old (48). A dexamethasone suppression test, looking for aldosterone suppression or blood pressure improvement, can indicate the diagnosis, as well as urine steroid profiling (20). Not all cases are hypokalaemic.
FH-2 shows incomplete penetrance, however mutations in the chloride channel gene, CLCN2, increase chloride conductance in the zona glomerulosa, which results in autonomous aldosterone synthesis (18). FH-3 clinically is similar to primary hyperaldosteronism, with significant adrenal hyperplasia or adenoma. This is due to mutations in the potassium channel gene KCNJ5 and should be indicated by aldosteronism presenting in children (<10 years old), and family history of adrenal hypertension. Genetic testing is available, but otherwise the clinical features are like primary hyperaldosteronism. FH-4 is caused by mutations in CACNA1H gene and was identified in children under 10 years old presenting with primary hyperaldosteronism (19).
Conditions associated with normotension or hypotension
Bartter and Gitelman syndromes
There are also rare conditions causing hypokalaemic metabolic alkalosis, associated with low or normal blood pressure. Perhaps the most well-known are the tubulopathies: Bartter and Gitelman syndromes, affecting approximately 1 in 100,000 and 25 in 100,000 respectively (49). These tubulopathies are autosomal recessive conditions, causing salt wasting and hence hyperaldosteronism; mimicking the effects of loop and thiazide diuretics with hypochloraemia also. Bartter syndrome affects the thick ascending limb of the loop of Henle, with loss of function of NKCC2, ROMK, Barttin, CLC-Kb or CLC-Ka; and associated with mutations in SLC12A1, KCNJ1, BSND (with sensorineural hearing loss), CLCNKB, and CLCKA respectively (50). Very rarely, mutations in MAGED2 have been reported in an X-linked presentation of antenatal Bartter syndrome, which affects the functioning and expression of NKCC2 and NCC (51). Gitelman syndrome is also characterised by hypocalciuria and hypomagnesaemia. A single mutation can result in a mild phenotype with fatigue and cramps. Gitelman syndrome reduces the function of the thiazide sensitive sodium-chloride symporter (NCC), located in the distal convoluted tubule, and is due to mutations of SLC12A3 (NCC) or CLCNKB (CLC-Kb) (52). It is worth noting that those with KCNJ1 mutations can present with transient hyperkalaemia; and CLCNKB and SLC12A3 hypomagnesaemia (24).
Gitelman syndrome can be distinguished from Bartter syndrome as it tends to demonstrate hypocalciuria (urine calcium:creatinine ratio <0.2 mol/moL) and dilute urine, whereas Bartter syndrome typically presents with hypocalcaemia and urine calcium:creatinine ratio >0.2 mol/moL. Gitelman is also more associated with hypomagnesaemia, but this can occur in Bartter too (27). Bartter syndrome presents in the antenatal period or milder phenotypes, e.g., classical Bartter, can present at any age but most commonly childhood (22). Gitelman syndrome is milder, but has very marked phenotypic variation, therefore severe cases do exist presenting earlier, however it can present later with some cases being relatively asymptomatic (22). Some cases of Gitelman can present with hypertension, adding to the possible confusion (22).
Clinical phenotype is inadequate to differentiate Bartter and Gitelman syndromes due to the overlap. However, a diuretic test or genetic testing can help confirm the diagnosis (22,28). The diuretic test works on the principle that a loop diuretic will have no effect in Bartter syndrome cases, as NKCC function is already disrupted, and thiazides have no effect in Gitelman as NCC is similarly affected (22,25). Urine sodium and chloride is measured on spot urines, 6 hours post administration, but there are various limitations (including the need to stop other therapies to perform the test), and the test is not always entirely diagnostic (22). Genetics, although probably the gold standard, can be complicated by the range of mutations described, but will continue to improve as techniques and knowledge improves (22).
It is worth noting that aminoglycoside antibiotics simulate a transient Bartter syndrome, causing metabolic alkalosis and hypomagnesaemia, sometimes known as acquired Bartter syndrome (22,53). Very rarely, acquired Gitelman syndrome has been described mostly secondary to Sjögren syndrome, and thought to be due to antibodies to NCC, as has Bartter syndrome (54).
SESAME
The seizures, sensorineural deafness, ataxia, mental retardation, and electrolyte imbalance syndrome or SESAME (also known as EAST, for epilepsy, ataxia, sensorineural deafness and tubulopathy, syndrome), is caused by a mutation in the KCNJ10 gene for an inward rectifying potassium channel (Kir4.1). This loss of function results in salt loss and hence stimulation of aldosterone release. Magnesium renal wasting and hypomagnesaemia also occurs, rather similar to Gitelman (55).
Autosomal dominant familial hypocalcaemia
Autosomal dominant familial hypocalcemia, affecting the calcium receptor gene CASR, causes a hypochloremic, hypokalaemic, metabolic alkalosis. This condition can be confused with Bartter syndrome as it presents with hypocalaemia (22). The dominant inheritance can help differentiate between the recessive Bartter syndrome, and PTH will also be inappropriately low (22).
Chloride losing diarrhoea
Other rare causes of hypochloremic alkalosis are chloride losing diarrhoea caused by CF or congenital chloride diarrhoea (CCD). CCD is caused by defects in the Cl−/HCO3− anion exchanger because of mutations in the sulphate permease transporter SLC26A3 gene. Normal faecal chloride concentration is 10–15 mmol/L, however this can increase to 90 mmol/L in CCD, but can be lower if very chloride deplete (56). Patients with CCD are alkalotic as HCO3− cannot be excreted (57). CF is caused by a mutation in the CF transmembrane conductance regulator (CFTR) gene, and is also linked with hyponatremia and hypochloraemia, due to hyperaldosteronism, with alkalosis, in order to replace the salt lost in sweat (58,59).
Dents disease
Dents disease is an X-linked disorder caused by mutations in CLCN5 (type 1) and OCRL1 (type 2), causing proteinuria, hypercalciuria, nephrolithiasis, and rickets, among other things (60). CLCN5 encodes the chloride channel protein 5 (CLCL-5) that exchanges H+ for Cl- ions in the proximal tubule. A few patients can manifest hypochloraemia, metabolic alkalosis, and hypokalaemia) (22,61).
Conclusions
The investigation of alkalosis can be challenging, with many conditions affecting the homeostasis. A correctly interpreted arterial blood gas can give clear indication of where the problem may have originated, and if that test is not available, we have presented how to work through a raised bicarbonate result. The diagnostic algorithms attached to this article will help the reader to systematically consider causes of alkalosis. However, we appreciate that each patient case is different and it may be appropriate to skip to steps later in the algorithms, refer to other algorithms (e.g., acidosis within this series), or even miss out steps entirely. These algorithms cannot replace specialist knowledge, experience and local guidelines. Instead, they should act as diagnostic aids when assessing alkalotic patients.
Acknowledgments
We would like to thank Professor Rousseau Gama, Laboratory of Clinical Chemistry and Hematology, University Hospital of Verona, Verona, Italy for inspiring and inviting us to prepare this paper. All figures were created with BioRender.com.
Funding: None.
Footnote
Provenance and Peer Review: This article was commissioned by the editorial office, Journal of Laboratory and Precision Medicine for the series “Investigative Algorithms in Laboratory Medicine—Electrolytes and Acid/Base”. The article has undergone external peer review.
Reporting Checklist: The authors have completed the Narrative Review reporting checklist. Available at https://jlpm.amegroups.com/article/view/10.21037/jlpm-22-8/rc
Conflicts of Interest: All authors have completed the ICMJE uniform disclosure form (available at https://jlpm.amegroups.com/article/view/10.21037/jlpm-22-8/coif). The series “Investigative Algorithms in Laboratory Medicine—Electrolytes and Acid/Base” was commissioned by the editorial office without any funding or sponsorship. ARS serves as Editor-in-Chief of Journal of Clinical and Experimental Dermatology. KS served as the unpaid Guest Editor of the series. The authors have no other conflicts of interest to declare.
Ethical Statement: The authors are accountable for all aspects of the work in ensuring that questions related to the accuracy or integrity of any part of the work are appropriately investigated and resolved.
Open Access Statement: This is an Open Access article distributed in accordance with the Creative Commons Attribution-NonCommercial-NoDerivs 4.0 International License (CC BY-NC-ND 4.0), which permits the non-commercial replication and distribution of the article with the strict proviso that no changes or edits are made and the original work is properly cited (including links to both the formal publication through the relevant DOI and the license). See: https://creativecommons.org/licenses/by-nc-nd/4.0/.
References
- Adrogué HJ, Madias NE. Secondary responses to altered acid-base status: the rules of engagement. J Am Soc Nephrol 2010;21:920-3. [Crossref] [PubMed]
- Stewart PA. Modern quantitative acid-base chemistry. Can J Physiol Pharmacol 1983;61:1444-61. [Crossref] [PubMed]
- Caprilli R, Frieri G, Latella G, et al. Faecal excretion of bicarbonate in ulcerative colitis. Digestion 1986;35:136-42. [Crossref] [PubMed]
- Dhondup T, Qian Q. Acid-Base and Electrolyte Disorders in Patients with and without Chronic Kidney Disease: An Update. Kidney Dis (Basel) 2017;3:136-48. [Crossref] [PubMed]
- Martinu T, Menzies D, Dial S. Re-evaluation of acid-base prediction rules in patients with chronic respiratory acidosis. Can Respir J 2003;10:311-5. [Crossref] [PubMed]
- Suarez N, Conway N, Pickett T. Panic-related hyperventilation resulting in hypophosphataemia and a high lactate. BMJ Case Rep 2013;2013:bcr2013009307. [Crossref] [PubMed]
- Edmondson JW, Brashear RE, Li TK. Tetany: quantitative interrelationships between calcium and alkalosis. Am J Physiol 1975;228:1082-6. [Crossref] [PubMed]
- Huijgen HJ, Soesan M, Sanders R, et al. Magnesium levels in critically ill patients. What should we measure? Am J Clin Pathol 2000;114:688-95. [Crossref] [PubMed]
- Korosi A, Kahn T, Kalb T, et al. Marked hyperlactatemia associated with severe alkalemia in a patient with thrombotic thrombocytopenic purpura. Am J Kidney Dis 2000;36:E6. [Crossref] [PubMed]
- Sasse SA, Berry RB, Nguyen TK, et al. Arterial blood gas changes during breath-holding from functional residual capacity. Chest 1996;110:958-64. [Crossref] [PubMed]
- O'Neill AV, Johnson DC. Transition from exercise to rest. Ventilatory and arterial blood gas responses. Chest 1991;99:1145-50. [Crossref] [PubMed]
- Davis MD, Walsh BK, Sittig SE, et al. AARC clinical practice guideline: blood gas analysis and hemoximetry: 2013. Respir Care 2013;58:1694-703. [Crossref] [PubMed]
- Goodwin NM, Schreiber MT. Effects of anticoagulants on acid-base and blood gas estimations. Crit Care Med 1979;7:473-4. [Crossref] [PubMed]
- Byrne AL, Bennett M, Chatterji R, et al. Peripheral venous and arterial blood gas analysis in adults: are they comparable? A systematic review and meta-analysis. Respirology 2014;19:168-75. [Crossref] [PubMed]
- Walkey AJ, Farber HW, O'Donnell C, et al. The accuracy of the central venous blood gas for acid-base monitoring. J Intensive Care Med 2010;25:104-10. [Crossref] [PubMed]
- Habibzadeh F, Yadollahie M. Temperature correction of the calculated alveolar oxygen tension. Anesthesiology 1998;89:1042. [Crossref] [PubMed]
- Sica DA, Carter B, Cushman W, et al. Thiazide and loop diuretics. J Clin Hypertens (Greenwich) 2011;13:639-43. [Crossref] [PubMed]
- Koch SM, Taylor RW. Chloride ion in intensive care medicine. Crit Care Med 1992;20:227-40. [Crossref] [PubMed]
- Palmer BF, Clegg DJ. The Use of Selected Urine Chemistries in the Diagnosis of Kidney Disorders. Clin J Am Soc Nephrol 2019;14:306-16. [Crossref] [PubMed]
- Bertulli C, Hureaux M, De Mutiis C, et al. A Rare Cause of Chronic Hypokalemia with Metabolic Alkalosis: Case Report and Differential Diagnosis. Children (Basel) 2020;7:212. [Crossref] [PubMed]
- Rutledge AC, Johnston A, Bailey D, et al. Survey of renin and aldosterone testing practices by Ontario laboratories - Providing insight into best practices. Pract Lab Med 2021;25:e00229. [Crossref] [PubMed]
- Cherniack RM. Physiologic diagnosis and function in asthma. Clin Chest Med 1995;16:567-81. [Crossref] [PubMed]
- Warren SE, Steinberg SM. Acid-base and electrolyte disturbances in anorexia nervosa. Am J Psychiatry 1979;136:415-8. [Crossref] [PubMed]
- Patel AM, Adeseun GA, Goldfarb S. Calcium-alkali syndrome in the modern era. Nutrients 2013;5:4880-93. [Crossref] [PubMed]
- Reiser IW, Ali S, Gotlieb V, et al. Hypercalcemia-Induced Hypokalemic Metabolic Alkalosis in a Multiple Myeloma Patient: The Risk of Furosemide Use. Case Rep Oncol 2015;8:389-93. [Crossref] [PubMed]
- Jdiaa SS, Walsh SB, Bockenhauer D, et al. The hypokalemia mystery: distinguishing Gitelman and Bartter syndromes from 'pseudo-Bartter syndrome'. Nephrol Dial Transplant 2021;37:29-30. [Crossref] [PubMed]
- Urwin S, Willows J, Sayer JA. The challenges of diagnosis and management of Gitelman syndrome. Clin Endocrinol (Oxf) 2020;92:3-10. [Crossref] [PubMed]
- Al Shibli A, Narchi H. Bartter and Gitelman syndromes: Spectrum of clinical manifestations caused by different mutations. World J Methodol 2015;5:55-61. [Crossref] [PubMed]
- Woywodt A, Herrmann A, Eisenberger U, et al. The tell-tale urinary chloride. Nephrol Dial Transplant 2001;16:1066-8. [Crossref] [PubMed]
- Stinebaugh BJ, Schloeder FX. Glucose-induced alkalosis in fasting subjects; relationship to renal bicarbonate reabsorption during fasting and refeeding. J Clin Investig 1972;51:1326-36. [Crossref] [PubMed]
- Li K, Xu Y. Citrate metabolism in blood transfusions and its relationship due to metabolic alkalosis and respiratory acidosis. Int J Clin Exp Med 2015;8:6578-84. [PubMed]
- Young WF, Calhoun DA, Lenders JWM, et al. Screening for endocrine hypertension: an endocrine society scientific statement. Endocrine Reviews 2017;38:103-22. [Crossref]
- Temple AR. Pathophysiology of aspirin overdosage toxicity, with implications for management. Pediatrics 1978;62:873-6. [Crossref] [PubMed]
- Terzano C, Di Stefano F, Conti V, et al. Mixed acid-base disorders, hydroelectrolyte imbalance and lactate production in hypercapnic respiratory failure: the role of noninvasive ventilation. PLoS One 2012;7:e35245. [Crossref] [PubMed]
- Abbassi-Ghanavati M, Greer LG, Cunningham FG. Pregnancy and laboratory studies: a reference table for clinicians. Obstet Gynecol 2009;114:1326-31. [Crossref] [PubMed]
- Teasdale S, Morton A. Changes in biochemical tests in pregnancy and their clinical significance. Obstet Med 2018;11:160-70. [Crossref] [PubMed]
- Enslow BT, Stockand JD, Berman JM. Liddle's syndrome mechanisms, diagnosis and management. Integr Blood Press Control 2019;12:13-22. [Crossref] [PubMed]
- Geller DS, Farhi A, Pinkerton N, et al. Activating mineralocorticoid receptor mutation in hypertension exacerbated by pregnancy. Science 2000;289:119-23. [Crossref] [PubMed]
- Pintavorn P, Munie S. A Case Report of Recurrent Hypokalemia During Pregnancies Associated With Nonaldosterone-Mediated Renal Potassium Loss. Can J Kidney Health Dis 2021;8:20543581211017424. [Crossref] [PubMed]
- Adeli K, Higgins V, Trajcevski K, et al. The Canadian laboratory initiative on pediatric reference intervals: A CALIPER white paper. Crit Rev Clin Lab Sci 2017;54:358-413. [Crossref] [PubMed]
- Mantero F, Palermo M, Petrelli MD, et al. Apparent mineralocorticoid excess: type I and type II. Steroids 1996;61:193-6. [Crossref] [PubMed]
- Rauh M. Steroid measurement with LC-MS/MS. Application examples in pediatrics. J Steroid Biochem Mol Biol 2010;121:520-7. [Crossref] [PubMed]
- Charmandari E, Kino T. Chrousos syndrome: a seminal report, a phylogenetic enigma and the clinical implications of glucocorticoid signalling changes. Eur J Clin Invest 2010;40:932-42. [Crossref] [PubMed]
- Marques P, Tufton N, Bhattacharya S, et al. Hypertension due to a deoxycorticosterone-secreting adrenal tumour diagnosed during pregnancy. Endocrinol Diabetes Metab Case Rep 2019;2019:18-0164. [Crossref] [PubMed]
- OMIM Entry - # 103900 - Hyperaldosteronism, familial, type 1;HALD1. Available online: https://www.omim.org/entry/103900 (accessed 06/01/2022).
- OMIM Entry - # 605635 - HYPERALDOSTERONISM, FAMILIAL, TYPE II; HALD2. Available online: https://www.omim.org/entry/605635 (accessed 6/1/2022).
- OMIM Entry - # 617027 - HYPERALDOSTERONISM, FAMILIAL, TYPE IV; HALD4. Available online: https://www.omim.org/entry/617027 (accessed 6/1/2022).
- Dluhy RG, Lifton RP. Glucocorticoid-remediable aldosteronism. J Clin Endocrinol Metab 1999;84:4341-4. [Crossref] [PubMed]
- Ji W, Foo JN, O'Roak BJ, et al. Rare independent mutations in renal salt handling genes contribute to blood pressure variation. Nat Genet 2008;40:592-9. [Crossref] [PubMed]
- Nuñez-Gonzalez L, Carrera N, Garcia-Gonzalez MA. Molecular Basis, Diagnostic Challenges and Therapeutic Approaches of Bartter and Gitelman Syndromes: A Primer for Clinicians. Int J Mol Sci 2021;22:11414. [Crossref] [PubMed]
- Laghmani K, Beck BB, Yang SS, et al. Polyhydramnios, Transient Antenatal Bartter's Syndrome, and MAGED2 Mutations. N Engl J Med 2016;374:1853-63. [Crossref] [PubMed]
- Walsh PR, Tse Y, Ashton E, et al. Clinical and diagnostic features of Bartter and Gitelman syndromes. Clin Kidney J 2018;11:302-9. [Crossref] [PubMed]
- Chou CL, Chen YH, Chau T, et al. Acquired bartter-like syndrome associated with gentamicin administration. Am J Med Sci 2005;329:144-9. [Crossref] [PubMed]
- Kim YK, Song HC, Kim YS, et al. Acquired gitelman syndrome. Electrolyte Blood Press 2009;7:5-8. [Crossref] [PubMed]
- OMIM Entry - # 612780 - Seizures, sensorineural deafness, ataxia, mental retardation, and electrolyte imbalance; sesames, Available online: https://www.omim.org/entry/612780 (accessed 7/1/22).
- Wedenoja S, Höglund P, Holmberg C. Review article: the clinical management of congenital chloride diarrhoea. Aliment Pharmacol Ther 2010;31:477-85. [Crossref] [PubMed]
- Kiela PR, Ghishan FK. Physiology of Intestinal Absorption and Secretion. Best Pract Res Clin Gastroenterol 2016;30:145-59. [Crossref] [PubMed]
- Bareil C, Bergougnoux A. CFTR gene variants, epidemiology and molecular pathology. Arch Pediatr 2020;27:eS8-eS12. [Crossref] [PubMed]
- Ozçelik U, Göçmen A, Kiper N, et al. Sodium chloride deficiency in cystic fibrosis patients. Eur J Pediatr 1994;153:829-31. [Crossref] [PubMed]
- Thakker RV. Pathogenesis of Dent's disease and related syndromes of X-linked nephrolithiasis. Kidney Int 2000;57:787-93. [Crossref] [PubMed]
- Li F, Yue Z, Xu T, et al. Dent Disease in Chinese Children and Findings from Heterozygous Mothers: Phenotypic Heterogeneity, Fetal Growth, and 10 Novel Mutations. J Pediatr 2016;174:204-210.e1. [Crossref] [PubMed]
Cite this article as: Allen GT, Shipman AR, Darragh-Hickey C, Flowers KC, Kaur S, Shipman KE. Investigative algorithms for disorders affecting alkalosis: a narrative review. J Lab Precis Med 2022;7:23.