Biomarkers and kidney diseases: a brief narrative review
Introduction
Current biomolecular identification techniques expose new aspects in the biological universe of the human body (1). Omics strategies through genomics, metabolomics, lipidomics and proteomics generate thousands of information that demand math resources to integrate and translate the meanings of multiple molecular patterns in the construction of modern biochemical knowledge of the interior environment.
The molecular scenarios designed with these tools contain biomarkers that, together or in isolation, are potentially capable of predicting or confirming diagnoses, guiding therapeutic strategies and predicting clinical outcomes. Biomarkers are endogenous molecules, detected qualitatively and/or quantitatively, which provide peculiar data for the identification of physiological or pathophysiological processes, as well as for the control of pharmacological responses. After more than a century restricted to the first biomarkers for the detection of kidney dysfunction—urea and creatinine (Cr), and kidney damage—proteinuria, nephrology now has a series of new molecular information and submits them to repeated validation so that they can be properly incorporated into clinical practice (2).
Kidney biomarkers can be classified according to the morphophysiological characteristics of the nephron, related both to the renal function (glomerular and tubular) and to the integrity of its endothelial or epithelial cells (Figure 1). The development of these biomarkers is not focused on the identification of a specific disease, but on the detection of a renal pathophysiological phenomenon, with variable complexities and etiologies. The absence or normal levels of certain biomarkers can also be clinically useful to define negative predictive values for various pathologies.
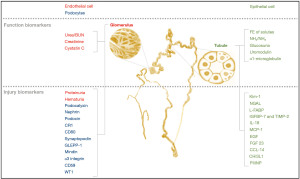
In this brief review, we describe old and new molecules, proposed as biomarkers and whose clinical insights have been demonstrated in the literature for various kidney diseases, with the aim of providing an update on the topic. We present the manuscript according to the Narrative Review reporting checklist (available at https://jlpm.amegroups.com/article/view/10.21037/jlpm-22-1/rc). The main glomerular and tubular biomarkers described in the literature so far are presented below.
Methods
We searched the PubMed database for clinical trial articles in English, published between January 1990 and December 2021, with the following terms: “AKI Biomarkers”, “Podocytopathy Biomarkers”, “Lupus Kidney Biomarkers”, “Diabetic Glomerulopathy Biomarkers”, “Diabetic Nephropathy Biomarkers”, “Tubular Renal Biomarkers”, “Renal Biomarkers” (Table 1).
Table 1
Items | Specification |
---|---|
Date of search (specified to date, month and year) | December 4 to 31, 2021 |
Databases and other sources searched | PubMed |
Search terms used (including MeSH and free text search terms and filters) | “AKI Biomarkers”; “Podocytopathy Biomarkers”; “Lupus Kidney Biomarkers”; “Diabetic Glomerulopathy Biomarkers”; “Diabetic Nephropathy Biomarkers”; “Tubular Renal Biomarkers”; “Renal Biomarkers” |
Timeframe | January 1990–December 2021 |
Inclusion criteria | English text; human clinical trial |
Selection process (who conducted the selection, whether it was conducted independently, how consensus was obtained, etc.) | Both authors selected and had consensus |
Glomerular function biomarkers
The quantification, in plasma and/or in urine, of endogenous low molecular weight molecules, which are freely filtrated by capillary endothelium, can be employed clinically for estimating glomerular filtration rate (GFR). Elevated serum levels of these biomarkers may indicate: (I) glomerulopenia, constitutional or acquired, as occur in chronic kidney disease (CKD), or (II) abrupt, intrinsic or reflex loss of glomerular function, which occurs in acute kidney injury (AKI). With its quantitative data, these molecules are part of the classic metabolomics of kidney failure, the metabolic signature from different past biological phenomena that compromised the functioning of the kidneys. As small molecules, these biomarkers are dialyzable and can be used as adequacy parameters for extrarenal clearance achieved by dialysis methods.
Urea/blood urea nitrogen (BUN)
Urea was isolated from human urine in 1797 (3) and was the first biomarker related to renal dysfunction. For over a century, the term uremia, meaning “urine in the blood”, has been used as a synonym for kidney failure. This 60-Da molecule is a nitrogenous product of protein metabolism, contains 2 nitrogen atoms, is freely filtered by the kidneys and between 40% to 70% of the filtered urea returns to the plasma through the tubular epithelium (4). Serum urea levels depend on: (I) hepatic urea generation rate; (II) protein intake; (III) urea tubular reabsorption intensity; (IV) use of some medications and (V) presence of gastrointestinal bleeding. Simple to dose and easy to interpret, urea has high sensitivity but low specificity for the diagnosis of kidney disease and is not used to quantify GFR.
Creatinine
Serum Cr, the most widely used biomarker in nephrology, is an endogenous component constantly released into the circulation from muscle creatine, at a daily rate between 1% and 2% of the body’s creatine content. Cr has 113 Da, does not bind to proteins, is freely filtered, not reabsorbed or metabolized in the kidney and was the first biomarker used for clinical quantification of GFR. About 25% of urinary Cr is secreted by renal tubules, influenced by drugs such as probenecid, penicillin, cimetidine and trimethoprim (5). Because Cr is related to muscle mass, its reference values vary between genders and age. Pharmacodynamic analysis estimated that the biological half-life of Cr in a healthy adult is around 4 h and is prolonged to 8 and 80 h with 50 and 5 percent of GFR respectively (6). As there is no linearity between serum Cr levels and GFR, math equations were developed for GFR estimation and this approach allowed clinical standardization of nomenclature for staging of nephropathies (7).
Cystatin C (Cys C)
Cys C is a 13-kDa cysteine proteinase inhibitor protein that has been incorporated as the latest biomarker to quantify and classify the stages of CKD (8). Cys C is synthesized in all nucleated cells, its serum levels do not depend on the musculature and do not suffer from age interference, showing some advantages for clinical use in relation to Cr. In the kidney, Cys C is freely filtered through the glomerular membrane, immediately reabsorbed in the proximal tubule (PT), where it is catabolized and, therefore, Cys C is not normally found in the urine nor returns to the circulation (9). Serum Cys C levels are affected by thyroid function, increase in hyperthyroidism and, unlike Cr, decrease in hypothyroidism. As serum levels of Cys C and Cr have the same hyperbolic relationship with GFR both biomarkers are employed in the same prediction equations for GFR (9). Cys C is a better as an option for patients with reduced muscle mass and degenerative muscle disease, where it is clearly a more accurate biomarker (8).
Glomerular injury biomarkers
Glomerular endothelial or podocyte/epithelial injury biomarkers can be detected in urine, qualitatively and quantitatively, and reveal an impairment of the glomerular capillary barrier, without necessarily affecting the GFR.
Proteinuria
Proteinuria can vary in quantity and quality and manifest as a transient or persistent elimination pattern. The urine of healthy individuals may contain a small amount of protein (<150 mg/day) which includes albumin, other plasma proteins (immunoglobulins, beta-2-microglobulin, etc.) and Tamm-Horsfall tubular protein (Table 2). Elevated proteinuria for more than 3 months, with or without a reduction in GFR, is sufficient to characterize the existence of CKD. Proteinuria is also a parameter used to assess therapeutic and pharmacological measures. Albuminuria is relevant for screening and prognosis in CKD, but it is also an independent risk factor for cardiovascular disease, end-stage kidney disease, stroke, and death. The nomenclature for the classification of albuminuria (7) is described in Tables 3. Classification A2 is associated with an increased risk of progression of kidney disease and cardiovascular events, and A3 is associated with lower renal survival (7).
Table 2
Proteinuria | Daily excretion | Qualitative characteristics |
---|---|---|
Nephrotic | >3.5 g | Heavy proteinuria |
Glomerular | 1–20 g | Plasma proteins (albumin mainly) |
Tubular | <2 g | LMW proteins (UMOD, RBP, α2 and β2 microglobulin) |
Overflow | Up to 20 g | Overexcretion of small proteins (MB, LCP) |
LMW, low molecular weight; UMOD, uromodulin; RBP, retinol binding protein; MB, myoglobulin; LCP, light chain protein.
Table 3
Albuminuria classification | Intensity | Daily excretion | Relationship, albumin/creatinine |
---|---|---|---|
A1 | Normal/light | <30 mg | <30 mg/g |
A2 | Moderate | 30–300 mg | 30–300 mg/g |
A3 | Serious | >300 mg | >300 mg/g |
Reagent strip tracking: + (30 mg/dL); ++ (100 mg/dL); +++ (300 mg/dL).
Hematuria
Blood cells do not cross the glomerular barrier in a significant amount and the presence of red blood cells in urine is always a concern, whether by macroscopic or microscopic detection, intermittently or continuously. Hematuria is defined as >10 erythrocytes per field (400×) (10). Urinary dipsticks are useful for screening and detecting the heme group of hemoglobin. At low density (<1.006), hypotonicity causes lysis of red cells and a test with positive strip for hemoglobin and paradoxically negative hematuria on microscopy can be possible. Glomerular hematuria is sometimes asymptomatic and symptom-rich hematuria usually originates along the urinary tract. Hematic casts make the diagnosis of active glomerulonephritis, which is usually accompanied by different degrees of proteinuria. Glomerular hematuria has a wide morphological variation and the identification of erythrocyte dysmorphism can be facilitated by phase-contrast microscopy, which allows the identification of acanthocytes and codocytes (11).
Glomerular podocytopathy biomarkers
Podocytes are epithelial cells and their foot processes cover the visceral epithelial layer on the exterior the glomerular basal membrane (GBM) surface. GBM integrity can be verified by non-invasive methods simply by taking a urine sample to identify and quantify podocyte-specific biomarkers. Podocyte urinary biomarkers reveal compromised structural integrity of the glomerulus and constitute clinically relevant information for glomerulopathies (12). Glomerular podocytopathies include minimal change disease (MCD), membranous glomerulopathy (MG), crescentic glomerulonephritis, collapsing glomerulopathy, focal segmental glomerulosclerosis (FSGS), diabetic nephropathy (DN), and lupus nephritis (LN). The following podocyte proteins can be detected in urine: podocalyxin, nephrin, podocin, complement receptor-1 (CR1), CD80, synaptopodin, GLEPP-1, mindin, alpha 3 integrin, CD59, and Wilms tumor protein 1 (WT1).
Podocalyxin
Podocalyxin in urine was related to podocyte injury (13,14) in patients with IgA nephropathy (IgAN) and Henoch-Schonlein purpura nephritis with a high sensitivity (88.4%) and specificity of 100% (15). In these patients, proteinuria levels are not able to reflect the severity of the disease, but urinary podocalyxin level does and represents an excellent non-invasive tool for the clinical management (16). Patients with severe diabetes mellitus albuminuria have more podocalyxin positive in the urine than diabetic patients with low microalbuminuria (17). Patients with FSGS that are excreters of podocalyxin in the urine had a fall of serum Cr in follow-up, indicting recovery (17). The connection between preeclampsia and podocytes has been enlightened by podocalyxin found in urine of women with preeclampsia and it can be used to diagnose this gestational pathology (18).
Nephrin
Nephrin is a 180-kDa transmembrane protein expressed in podocytes that was first identified in children with congenital nephrotic syndrome of the Finnish type (NPHS1) (19). Podocytopathies result in the detection of nephrin in the urine and nephrinuria is an early biomarker that showed positive correlation with proteinuria and severity of podocyte injury (20). Nephrinuria is elevated in DN and may play a role in clinical stratification of these patients (21) been a robust biomarker of preclinical DN (22). In adults with lupus or chronic glomerulonephritis, urine nephrin levels were positively correlated with disease severity and proteinuria (23). In obstetric patients, nephrinuria showed high sensitivity and specificity in pre-eclampsia detection (24).
Podocin
Podocin is a 42-kDa membrane-associated protein expressed by the NPHS2 gene which is mutated in some patients with steroid-resistant autosomal recessive nephrotic syndrome (25). The name podocin derives from the location of the protein, which is the podocyte membrane, where it plays a role in mediating cell signaling by interacting with nephrin (26). Podocin has greater diagnostic accuracy as a biomarker for preeclampsia podocytopathy compared to podocalyxin and nephrin (27). Urinary podocin and nephrin mRNA presented correlated with the rate of decline in GFR in patients with lupus, ND, IgAN, MCD, and MN (28).
CR1
CR1, also named CD35 or C3bR, is a podocyte membrane receptor with 200 kDa, expressed in both fetal and mature podocytes. CR1 expression on podocytes may contribute to complement-mediated damage in the kidney and CR1 urine levels have been studied in patients with lupus active glomerulonephritis and nephrotic syndrome (29,30).
CD80
CD80, also named B7-1, CD80 is a 53 kDa membrane podocyte protein that is also expressed in renal tubules, and has an immune role in T-lymphocyte activation. Promising data shows that urinary levels of CD80 in patients with relapsed MCD were significantly greater when compared to those in patients with MCD in remission and other glomerulopathies (31,32). Urinary CD80 is not affected by serum CD80 suggesting that it can also be derived from tubular epithelium, its specificity as a biomarker for podocytopathy remains to be confirmed.
Synaptopodin
Synaptopodin, also called 44 KDa podocyte protein, it is a podocyte actin-binding protein that regulates the cytoskeleton, which is also expressed in the central nervous system (33,34). Synaptopodin is a marker used in in vitro cell culture to control podocyte phenotypic differentiation. This protein has a renoprotective effect linked on its property to maintain and restore the podocyte’s cytoskeleton after injury. Data from patients with DN (35) showed that urinary synaptopodin mRNA significantly correlated with the number of glomerular podocytes seen on histological examination, suggesting that urinary mRNA could be used to monitor disease progression (36). The same application would be proposed to follow the progression in LN, IgAN, MCD and MN (36), but it does not seem to be a good biomarker for preeclampsia (37).
Glomerular epithelial protein 1 (GLEPP-1)
This was the first protein characterized in podocytes and named GLEPP-1 (38). It is an apical membrane protein also called protein tyrosine phosphatase receptor type-0 (PTPR0) with 132 kDa and a large extracellular domain participating in glomerular barrier integrity. Mutations with phenotypic changes of GLEPP-1 podocytes have been shown in patients with idiopathic nephrotic syndrome, steroid resistant subtype (39). GLEPP-1 is a potential biomarker that has been used in experimental models, but to date there are no relevant data in human glomerulopathy.
Mindin
Mindin or spondin-2 is a secreted extracellular matrix protein expressed in glomerular podocytes that can be detected and quantified in urine. This protein may have a role as a recruiter of inflammatory cells in the pathogenesis of glomerular lesions and functions as an opsonin for the phagocytosis of bacteria (40). One series showed that diabetic patients had increased urinary Mindin in proportion to their respective levels of albuminuria and the amount of urinary Mindin increased with the progression of DN. When compared with the same levels of albuminuria, lower values of urinary Mindin were found in patients with IgAN. Mindin seems to be related to podocyte injury in patients with type 2 diabetes and DN (41).
α3 integrin (Intα3)
Intα3 is a heterodimeric, podocyte basal-membrane surface protein responsible for the attachment of the foot processes maturation to the underlying GBM. Integrins are transmembrane receptors consisting of αβ subunits, which exhibit different binding properties and mediate cell adhesion and migration, cell-to-cell communication, and activation of intracellular signaling; Integrin α3β1 is the most highly expressed integrin in the kidney, is found in both the glomerulus and the tubules and is the major integrin involved in podocyte stability. In vivo study showed that the mutation of murine integrin α3 gene caused abnormal kidney and lung development (42). Children carrying deletion or missense homozygote mutations have kidneys with atrophic glomeruli, FSGS, diffuse interstitial fibrosis, tubular atrophy, proteinuria, and loss and immaturity of the tubules (43). The latency associated peptide-transforming growth factor β (TGF-β) and soluble urokinase plasminogen activator receptor (suPAR) was found as the novel ligands of integrins that contribute to renal interstitial fibrosis and FSGS (44). It has been proposed that suPAR binds primarily to β3 integrin on the surface of podocytes via a tripartite complex of suPAR-APOL1-β3 integrin risk variants (45) and more studies will be needed to fully understand the role of Intα3 in the pathophysiology of human diseases.
CD59
CD59, also called protectin or 20-kDa homologous restriction factor—HRF20, is a glycosylphosphatidylinositol anchored membrane bound protein that regulates the formation of the complement complex in the immune cascade. CD59 would be able to inhibit the complement mediated immune response to subepithelial immune complex deposition (46). CD59 concentration in the urine of patients with MN was higher in comparison to healthy controls and patients with DN. The urinary CD59 levels did not correlate to serum Cr, urinary protein concentration, or duration of disease (47).
WT1
This protein is a pivotal transcription factor exclusively expressed in glomerular podocytes. WT1 has been shown to be required for normal embryonic kidney development as well as the maintenance of the differentiated state of podocytes in adult kidneys (48). Urinary WT1 mRNA is significantly increased in patients with DN and chronic glomerulonephritis but undetectable in normal volunteers (49). WT1 is constitutively expressed in podocytes from healthy adults and is decreased in FSGS biopsies (49). Urinary exosomal WT1 was detected in some patients with active childhood nephrotic syndrome (50). In a mouse model of collapsing glomerulopathy, urinary exosomal WT1 was predicted for disease onset before proteinuria (51). Urinary WT1 represents a promising non-invasive biomarker for detecting podocyte lesions, monitoring disease progression and predicting therapeutic outcomes.
Tubular function biomarkers
Fractional excretion of solutes (FeS)
Fractional excretion (Fe) of any plasma filtered solute is a simple test used to assess tubular function that does not require timed urine collection and uses spot samples of urine and plasma. The Fe consists of calculating the percentage of filtered solute that is excreted in the urine, using respective simultaneous values of solutes and Cr. The results are computed: FeS = [(urinary solutes/plasma solutes)/(urinary Cr/plasma Cr)] ×100 (%). Fe is based on physiological principles that involve the reabsorption of different solutes by the tubular epithelium and reveals how much of the filtered solute has been excreted in the urine. The test is useful to assess FeNa (sodium) and FeU (urea) in cases of uremia and hypovolemia. The damaged tubule is incapable of reabsorbing the filtered elements in adequate amounts and FeNa <1 and FeU <35 are indicative of the integrity of the tubular function, pointing to volume depletion and probable pre-renal AKI. Different factors interfere with the excretion fractions, including the use of diuretics that increase FeNa but not FeU, making the latter a more reliable test for the etiological interpretation of AKI (52). Other exceptions with non-pre-renal AKI FeNa <1 occur when AKI due to acute tubular necrosis overlap in patients with previous perfusion disorder, as occurs in hepatorenal syndromes, heart failure and major burns. Patients with CKD adaptation mechanisms cause FeNa to increase inversely to the GFR values. Different hereditary and acquired tubulopathies can alter the excretion fractions of different solutes (such as phosphorus, calcium, uric acid, chlorine, bicarbonate, magnesium, etc.) and must be interpreted in light of physiological and pathophysiological circumstances.
Ammonium (NH4)/ammonia (NH3)
Physiologically, acidosis or acid load stimulates ammoniagenesis (NH3) in PT cells, with subsequent protonation in the lumen. Therefore, the low urinary NH4 may reflect different dysfunction of tubular cells. The main mechanism of urinary acidification is the excretion of NH4 and different pathological conditions are able to affect this tubular function. CKD causes the inability to adapt urinary ammoniagenesis and NH4 excretion to acid load. Urine ammonia was associated with clinical and pathological features of chronicity and tubulointerstitial disease activity among patients with LN and is a tool to help define the chronicity index and estimated GFR (eGFR) at biopsy (53). In AKI, low urine NH4 may reflect tubular decreased ability to generate NH4, that also can mean low hydrogen secretion and metabolic acidosis. The association of reduced urinary acidification with the progression of diabetic kidney disease in type 2 diabetes has already been demonstrated. Patients who form idiopathic uric acid calculi have an impaired renal excretory NH4 response when exposed to an acute acid load, and this defective tubular response appears to contribute to the pathogenesis of nephrolithiasis (54).
Glucosuria
Under physiological conditions there is no urinary excretion of glucose. Around 1.5 kg of glucose are filtered daily and the entire amount is reabsorbed by the renal tubules. Virtually all glucose is recovered in the PT by sodium-glucose cotransporters (SGLT). Glycosuria is a biomarker for the following conditions: (I) hyperglycemia with a filtered glucose load greater than the reabsorption capacity; (II) hereditary or acquired tubulopathies (e.g., Fanconi syndrome); (III) use of glucosuric drugs, especially SGLT2 inhibitors (gliflozines) (55).
Uromodulin (UMOD)
UMDO also known as Tamm-Horsfall protein, is a 95-kDa glycoprotein expressed in the thick portion of the ascending loop of Henle (TAL). It is the most abundant protein in urine, excreting about 50 to 150 mg/day, which can also be found in the composition of tubular casts. UMOD is proposed as one of the new biomarkers for AKI, given the negative association between its urinary concentration and the development of AKI, especially in pediatric patients (56). In AKI, UMOD seems to protect the tissue by reducing inflammatory events in the external medullary zone, an effect generated through Toll-like receptor 4 (TLR4) receptors (57). UMOD is a biomarker of tubular function in Fabry nephropathy (58) and is also able to predict tubulointerstitial inflammation in patients with active LN (59). Among deceased donor transplant recipients, those with AKI had lower levels of UMOD within the tubules. The presence of UMOD is closely related to the development and functional maturation of the loop of Henle (60). Fetuses with prenatal renal failure tend to have lower levels of UMOD, and their elevated levels in amniotic fluid or fetal urine are suggestive of preserved renal function in the fetus (61).
α1 microglobulin (α1-M)
α1-M is a 33-kDa protein synthesized in the liver and freely filtered by glomeruli, with immediate reabsorption by PT cells, where it is catabolized. Under normal conditions only a small amount of filtered α1-M appears in the urine. Because it is stable in the pathophysiological range of urine pH, the protein is used as a biomarker to reveal renal tubular dysfunction as occur in sepsis AKI (62), after heart surgery (63) and even detection of kidney damage after neonatal asphyxia (64). Urinary α1-M excretion in CKD is associated with faster disease progression and higher mortality, and urine α1-M levels >15 mg/g Cr may indicate PT damage (62). Urinary measurement of α1-M can be useful as a screening method for tubular proteinuria of different causes. More recently, elevated tubular proteinuria has been performed in patients hospitalized with COVID-19 and the high amount of α1-M excreted in urine has been associated as a biomarker of mortality in adjusted models (65).
Tubular injury biomarkers
Acute kidney injury molecule-1 (Kim-1)
Kim-1 is a 38.7-kDa transmembrane glycoprotein expressed in PT cells that is involved in the phagocytosis of epithelial cells subjected to ischemic or toxic injury (66,67). Quantitative detection of urinary Kim-1 increases linearly with age, even in healthy individuals, with the highest levels seen in men. Urinary and plasma Kim-1 values show that Kim-1 is an early biomarker of PT cell proliferation and regeneration, with significant sensitivity and specificity for AKI, both in human studies and experimental series (68). In cases of post-cardiac surgery AKI, Kim-1 levels significantly increase between 2 and 24 hours postoperatively (68,69). Kim-1 has been used as a biomarker for the study of drug nephrotoxicity (70). In addition to AKI, Kim-1 is a useful tool for detecting CKD progression and for guiding therapeutic interventions in patients at risk for developing CKD. High levels of Kim-1 are related to the stages of severity of CKD and are altered in cases of proteinuria and inflammatory kidney disease (71). For use in clinical practice new tests provide semi-quantitative results that allow the detection of urinary Kim-1 levels in 15 minutes (72).
Neutrophil gelatinase-associated lipocalin (NGAL)
NGAL is a 25-kDa protein first described in neutrophil granules, but also synthesized in various tissues, such as kidney, lung and digestive tract, after epithelial damage. Circulating molecules are filtered by the glomerulus and reabsorbed in the PT (73,74). The physiological production of NGAL increases with age and is greater in women and the main sites of NGAL generation in the kidney are the ascending loop of Henle and the collecting duct. In AKI, the decrease in tubular reabsorption promotes early urinary detection of NGAL, which rises in urine and plasma between 3 hours and 5 days after the initial injury. Increased NGAL expression can also occur due to bacterial infections, systemic inflammatory response syndrome and chronic and non-infectious systemic diseases. Therefore, inflammation is a confounding factor for the use of NGAL as an AKI biomarker, especially in critically ill patients with sepsis (75). In adult patients with sepsis, urinary NGAL alone was not an adequate predictive parameter to be used for the prevention of AKI, as well as for the need for Renal replacement therapy and for mortality within 90 days. However, NGAL proved to be a good predictive biomarker for AKI after cardiac surgery in adults (76) and vancomycin nephrotoxicity (77).
Liver fatty acid binding protein (L-FABP)
Liver-type fatty acid (FA) binding protein is a 14-kDa intracellular protein expressed in various tissues under physiological and pathophysiological conditions, including the PT and pars recta cells of the human nephron. L-FABP plays a relevant role in tissue injury and repair mechanisms and urinary L-FABP levels can identify the occurrence and severity of various kidney diseases. Physiologically, L-FABP is critical for FA homeostasis and is a promising biomarker to be used in different nephropathies (78). The protein binds to FA in the cytoplasm and transports them to the mitochondria or peroxisomes, where they are metabolized and provide energy for the work of the tubular epithelial cells. Urinary L-FABP is an early biomarker for detection and prognosis of acute stress-generating events and tubulointerstitial damage such as ischemia, post-cardiac surgery, post-renal transplantation, radiocontrast nephropathies, nephrotoxicity and sepsis. Urinary L-FABP may also reveal tubular lesions in CKD, such as nephrotic syndrome, DN, and glomerulopathies. These situations lead to excess FA within the tubular cells due to glomerular losses of FA-binding albumin. Under these conditions, it is believed that the increased expression of the L-FABP gene in the kidney is a protective response to facilitate the metabolism of FA and inhibit inflammatory phenomena. The greatest technical difficulty for the use of urinary L-FABP in clinical decisions is due to the long time required for its quantification, a barrier that has been overcome by the development of rapid quantitative tests (79) by chemiluminescence, and reagent kits of semiquantitative results (80). Urinary L-FABP is a diagnostic and predictive biomarker of AKI in critically ill patients.
Insulin-like growth factor binding protein-7 (IGFBP7) and tissue inhibitor of metalloproteinase 2 (TIMP-2)
In response to ischemic or septic AKI, the renal epithelial cells suffer from blockage of their cell cycles in the G1 phase. The two biomarkers IGFBP7 with 29 kDa and TIMP-2 with 21 kDa are involved in this stage of the cell cycle and have been studied together through the product of their respective urinary concentrations. In healthy individuals, the product of the values of these biomarkers did not differ significantly between men and women. The data suffer interference from diabetes, hyperbilirubinemia and anemia, which can induce false positive results (81). Therefore, these tests are complementary exams and the interpretations must be associated with the clinical picture that generated the suspicion of renal impairment. Among more than 300 biomarker candidates studied in critically ill patients, the product (IGFBP7) × (TIMP-2) proved to be the best predictive data for AKI 12 hours after the insult, and the simultaneous use of these urinary biomarkers may be useful in the intensive care unit (ICU) in patients at risk of developing AKI (82). One of the limitations for interpretation is not predicting the evolution of the already established AKI, as the duration and evolutionary kinetics of these two urinary biomarkers are not yet known.
Interleukin-18 (IL-18)
IL-18 is a 24-kDa proinflammatory cytokine, originally identified as an interferon-γ-inducing proinflammatory factor that regulates innate and adaptive immunity. The inactive precursor of IL-18 is synthesized in several organs, including the epithelial cells of the PT and renal collecting ducts (83). Inflammatory processes produce caspase 1 which activates IL-18 which is eliminated in the urine. Urinary IL-18 has been investigated as a biomarker of AKI (84). Detected 6 hours after ischemic injury, it can reach 25 times the normal value in 12 hours and IL-18 anticipates the diagnosis of AKI based on Cr in 24 to 48 hours. The best predictive evidence with IL-18 was observed in series of pediatric cardiac surgeries in which urinary levels increased early and significantly in patients who developed AKI (85). Urinary IL-18 is also increased in kidney transplant patients who have delayed graft function (86).
Monocyte chemotactic peptide-1 (MCP-1)
MCP-1, also known as C-C motif chemokine ligand 2 (CCL2), is produced by several different cells and is responsible for the migration of monocytes and macrophages to tissues, as part of the inflammatory reaction. Urinary MCP-1 has been studied as a biomarker of inflammatory processes that occur after AKI resulting from ischemia or toxicity. The use of MCP-1 blockers in experimental models of kidney disease has produced beneficial effects and MCP-1 inhibition is a proposed therapeutic strategy for patients with inflammatory kidney disease (87). Urinary MCP-1 and epidermal growth factor (EGF) have also been studied in the context of diabetic kidney disease, and both have been significantly associated with disease progression (88). In addition to the importance of the isolated values, the lower EGF/MCP-1 ratio was predictive of renal function decline in diabetes mellitus, IgAN, and several other glomerular diseases (89). These biomarkers appear to be useful in detecting the incidence or progression of CKD in patients undergoing cardiac surgery and help to stratify the long-term risk of CKD in other recognized clinical settings of kidney injury (89).
EGF
EGF is a 6-kDa mediator that regulates and stimulates the proliferation, migration and differentiation of mesenchymal cells, especially in the epithelia. EGF expression occurs mainly in PT cells, where it is transiently decreased after an ischemic insult and has been investigated as a biomarker of renal pathophysiological events (90). Clinical evidence shows that urinary EGF levels are reduced in patients with AKI (90). Experimentally, activation of EGF receptors (EGFR) in renal tissue is detected 5 to 30 minutes after ischemia/reperfusion, followed by the generation of free radicals and a reduction in the level of urinary EGF (91). The EGFR stimulates the dedifferentiation and proliferation of cells that survive injuries. Functional studies have shown that conditioned EGFR deletion accelerates renal tissue repair (92). Activation of the EGF/EGFR signaling pathway appears to be a promising strategy for the treatment of AKI and for recovery after AKI. As EGFR activation is associated with cell cycle arrest in the G2/M phase and may stimulate renal fibrogenesis, the therapeutic use of exogenous EGF may not be an adequate strategy for long-term treatment as EGFR may have ambiguous effect regulating both repair and fibrosis. Elevated urinary EGF was associated with greater recovery of renal function within 7 days and lower mortality within 3 months. Lower urinary EGF levels are associated with greater interstitial fibrosis and tubular atrophy (93). Higher EGF levels are associated with a better therapeutic response and remission in several glomerular diseases (94).
Fibroblast growth factor-23 (FGF-23)
FGF-23, also called phosphatonin, is a hormone that regulates the renal excretion of phosphorus that has been shown to be an early biomarker for AKI and the prognosis of adverse outcomes in patients with established AKI (95). FGF-23, with 28 kDa, is significantly increased in the urine and blood of patients with AKI and in different experimental models, such as ischemia/renal reperfusion and folic acid-induced AKI and rhabdomyolysis. Clinical evidence confirms that circulating levels of FGF-23 significantly increase in infants, children, adults and the elderly with AKI (96). Decreased clearance of FGF-23 in patients with AKI also contributes to increased circulating levels of FGF-23. Several studies have revealed mechanisms underlying the upregulation of FGF-23 in AKI, whose quantitative changes make it a robust candidate for a new predictive and prognostic biomarker to be used in patients with AKI (96,97).
C-C motif chemokine ligand 14 (CCL14)
Recently, the RUBY study (98) described urinary CCL-14 as the best performing cytokine among biomarkers predictive of AKI severity and persistence. Elevated urinary CCL14 levels are correlated with the risk of non-recovery of renal function. The mechanisms by which CCL1 interferes in the persistence of kidney damage are not yet known. CCL14 is a member of the family of small molecule chemokines that were initially linked to leukocyte chemotaxis and are implicated in tissue damage and repair processes (99). The level of urinary CCL14 seems to reflect the extent of the renal parenchymal damage. CCL14 is an important chemokine for monocyte/macrophage recruitment and is associated with pro-inflammatory chemotaxis in a variety of diseases including rheumatoid arthritis, multiple sclerosis and lupus. CCL14 is not expressed in mice and rats and little is known about the role and nature of CCL14 in AKI. CCL14 has a potential role as an inflammatory biomarker in identifying the risk of end-stage CKD in diabetic patients.
Chitinase 3 like-1 (CHI3L1)
CHI3L1, also known as YKL-40, is a 40-kDa glycoprotein secreted by several different cells. Urinary proteomics of experimental models revealed that CHI3L1 protects tubular cells from apoptosis and improves the survival of animals submitted to the ischemia/reperfusion AKI model (98). The molecule began to be investigated in patients at risk for AKI undergoing cardiac surgery and kidney transplantation. The combination of serum and urinary CHI3L1 was a good predictor of AKI associated with elective cardiac surgery at Kidney Disease: Improving Global Outcomes (KDIGO) stage ≥2 12 hours postoperatively and urinary CHI3L1 was associated with staging and death from AKI during hospitalization (100). CHI3L1 represents an AKI stage predictive biomarker ≥ KDIGO 2 in critically ill adult patients (101) and elevated urinary CHI3L1 is considered a promising biomarker for human septic AKI diagnostic (102). Specifically in malaria-associated AKI, CHI3L1 has been shown to be a robust new AKI biomarker with independent risk for mortality (103). In CKD, in baseline eGFR-adjusted models and albuminuria urinary CHI3L1 is associated with increased risk progression and was related to renal fibrosis and activation of myofibroblasts. In interstitial cystitis, serum and urine CHI3L1 levels are suggested as non-invasive biomarkers for the assessment of bladder fibrogenesis. Elevated serum concentrations of CHI3L1 have been reported in patients with type 2 diabetes, with certain solid tumors, other inflammatory conditions and in liver fibrosis (104,105).
Type III aminoterminal propeptide procollagen (PIIINP)
Renal fibrosis results from the deposition of various components of the extracellular matrix, including type III collagen. The degree of tubulointerstitial fibrosis present in renal biopsies is strongly associated with the progressive loss of GFR in CKD of different etiologies. Series of renal biopsies revealed that urinary PIIINP concentrations, with 44 kDa, correlated with the histological severity of tubulointerstitial fibrosis (106,107). As it is a small molecule, capable of glomerular filtration, the quantitative assessment of PIIINP in blood and urine and the PIIINP/Cr ratio in urine aim to validate this biomarker as an assessment tool for parenchymal fibrosis and renal function decline. Unlike blood measurements, urine PIIINP concentrations are associated with progression of CKD in the adult population, independently of baseline GFR conditions, albumin/Cr ratio and diagnosis of CKD (108). Urine PIIINP has the advantage of being a non-invasive method that presents itself as an independent factor associated with all-cause mortality in the elderly and emerges as a promising biomarker to assess the intensity of renal fibrosis and to monitor the evolution of renal grafts (109). Unlike other biomarkers, PIIINP does not reveal acute lesions of tubular cells and provides a predictive characteristic for the progression of nephropathies in the long term.
Conclusions
In summary, advances in biotechnology have made available a variety of biomarker molecules for kidney events. These molecules are of potential application in clinical practice, both for function evaluation and for the detection of pathophysiological processes present in different parts of the nephron. The development of these biomarkers is not focused on the identification of a specific disease, but on the detection of a kidney pathophysiological phenomenon, with variable complexities and etiologies.
Acknowledgments
Funding: None.
Footnote
Reporting Checklist: The authors have completed the Narrative Review reporting checklist. Available at https://jlpm.amegroups.com/article/view/10.21037/jlpm-22-1/rc
Conflicts of Interest: Both authors have completed the ICMJE uniform disclosure form (available at https://jlpm.amegroups.com/article/view/10.21037/jlpm-22-1/coif). The authors have no conflicts of interest to declare.
Ethical Statement: The authors are accountable for all aspects of the work in ensuring that questions related to the accuracy or integrity of any part of the work are appropriately investigated and resolved.
Open Access Statement: This is an Open Access article distributed in accordance with the Creative Commons Attribution-NonCommercial-NoDerivs 4.0 International License (CC BY-NC-ND 4.0), which permits the non-commercial replication and distribution of the article with the strict proviso that no changes or edits are made and the original work is properly cited (including links to both the formal publication through the relevant DOI and the license). See: https://creativecommons.org/licenses/by-nc-nd/4.0/.
References
- Wang JH, Byun J, Pennathur S. Analytical approaches to metabolomics and applications to systems biology. Semin Nephrol 2010;30:500-11. [Crossref] [PubMed]
- Ix JH, Shlipak MG. The Promise of Tubule Biomarkers in Kidney Disease: A Review. Am J Kidney Dis 2021;78:719-27. [Crossref] [PubMed]
- Fourcroy A, Vauquelin N. Memoire pour servir a l'histoire naturelle chimique et médicale de l'urine humaine. In: Mémoires de l'Institut National des Sciences et Arts. Paris: Baudouin, 1797:431.
- Lassiter WE, Gottschalk CW, Mylle M. Micropuncture study of net transtubular movement of water and urea in nondiuretic mammalian kidney. Am J Physiol 1961;200:1139-47. [Crossref] [PubMed]
- Eisner C, Faulhaber-Walter R, Wang Y, et al. Major contribution of tubular secretion to creatinine clearance in mice. Kidney Int 2010;77:519-26. [Crossref] [PubMed]
- Chiou WL, Hsu FH. Pharmacokinetics of creatinine in man and its implications in the monitoring of renal function and in dosage regimen modifications in patients with renal insufficiency. J Clin Pharmacol 1975;15:427-34. [Crossref] [PubMed]
- KDIGO 2012 Clinical Practice Guideline for the Evaluation and Management of Chronic Kidney Disease. Kidney International Supplements 2013;3:1-150.
- Randers E, Erlandsen EJ. Serum cystatin C as an endogenous marker of the renal function--a review. Clin Chem Lab Med 1999;37:389-95. [Crossref] [PubMed]
- van Deventer HE, Paiker JE, Katz IJ, et al. A comparison of cystatin C- and creatinine-based prediction equations for the estimation of glomerular filtration rate in black South Africans. Nephrol Dial Transplant 2011;26:1553-8. [Crossref] [PubMed]
- Davis R, Jones JS, Barocas DA, et al. Diagnosis, evaluation and follow-up of asymptomatic microhematuria (AMH) in adults: AUA guideline. J Urol 2012;188:2473-81. [Crossref] [PubMed]
- Fairley KF, Birch DF. Hematuria: a simple method for identifying glomerular bleeding. Kidney Int 1982;21:105-8. [Crossref] [PubMed]
- Sekulic M, Pichler Sekulic S. A compendium of urinary biomarkers indicative of glomerular podocytopathy. Patholog Res Int 2013;2013:782395. [Crossref] [PubMed]
- Hara M, Yamagata K, Tomino Y, et al. Urinary podocalyxin is an early marker for podocyte injury in patients with diabetes: establishment of a highly sensitive ELISA to detect urinary podocalyxin. Diabetologia 2012;55:2913-9. [Crossref] [PubMed]
- Hara M, Yanagihara T, Itoh M, et al. Immunohistochemical and urinary markers of podocyte injury. Pediatr Nephrol 1998;12:43-8. [Crossref] [PubMed]
- Asao R, Asanuma K, Kodama F, et al. Relationships between levels of urinary podocalyxin, number of urinary podocytes, and histologic injury in adult patients with IgA nephropathy. Clin J Am Soc Nephrol 2012;7:1385-93. [Crossref] [PubMed]
- Nakamura T, Ushiyama C, Suzuki S, et al. Urinary excretion of podocytes in patients with diabetic nephropathy. Nephrol Dial Transplant 2000;15:1379-83. [Crossref] [PubMed]
- Mueller-Deile J, Kümpers P, Achenbach J, et al. Podocalyxin-positive glomerular epithelial cells in urine correlate with a positive outcome in FSGS. J Nephrol 2012;25:802-9. [Crossref] [PubMed]
- Karumanchi SA, Lindheimer MD. Preeclampsia and the kidney: footprints in the urine. Am J Obstet Gynecol 2007;196:287-8. [Crossref] [PubMed]
- Kestilä M, Lenkkeri U, Männikkö M, et al. Positionally cloned gene for a novel glomerular protein--nephrin--is mutated in congenital nephrotic syndrome. Mol Cell 1998;1:575-82. [Crossref] [PubMed]
- do Nascimento JF, Canani LH, Gerchman F, et al. Messenger RNA levels of podocyte-associated proteins in subjects with different degrees of glucose tolerance with or without nephropathy. BMC Nephrol 2013;14:214. [Crossref] [PubMed]
- Jim B, Ghanta M, Qipo A, et al. Dysregulated nephrin in diabetic nephropathy of type 2 diabetes: a cross sectional study. PLoS One 2012;7:e36041. [Crossref] [PubMed]
- Wang G, Lai FM, Lai KB, et al. Messenger RNA expression of podocyte-associated molecules in the urinary sediment of patients with diabetic nephropathy. Nephron Clin Pract 2007;106:c169-79. [Crossref] [PubMed]
- Chebotareva NV, Bobkova IN, Kozlovskaia LV, et al. Estimation of podocyte dysfunction by nephrinuria severity in proteinuric forms of chronic glomerulonephritis. Ter Arkh 2011;83:18-23. [PubMed]
- Jim B, Mehta S, Qipo A, et al. A comparison of podocyturia, albuminuria and nephrinuria in predicting the development of preeclampsia: a prospective study. PLoS One 2014;9:e101445. [Crossref] [PubMed]
- Boute N, Gribouval O, Roselli S, et al. NPHS2, encoding the glomerular protein podocin, is mutated in autosomal recessive steroid-resistant nephrotic syndrome. Nat Genet 2000;24:349-54. [Crossref] [PubMed]
- Huber TB, Kottgen M, Schilling B, et al. Interaction with podocin facilitates nephrin signaling. J Biol Chem 2001;276:41543-6. [Crossref] [PubMed]
- Kerley RN, McCarthy C. Biomarkers of glomerular dysfunction in pre-eclampsia - A systematic review. Pregnancy Hypertens 2018;14:265-72. [Crossref] [PubMed]
- Szeto CC, Lai KB, Chow KM, et al. Messenger RNA expression of glomerular podocyte markers in the urinary sediment of acquired proteinuric diseases. Clin Chim Acta 2005;361:182-90. [Crossref] [PubMed]
- Pascual M, Steiger G, Sadallah S, et al. Identification of membrane-bound CR1 (CD35) in human urine: evidence for its release by glomerular podocytes. J Exp Med 1994;179:889-99. [Crossref] [PubMed]
- Khera R, Das N. Complement Receptor 1: disease associations and therapeutic implications. Mol Immunol 2009;46:761-72. [Crossref] [PubMed]
- Hara M, Yanagihara T, Kihara I, et al. Apical cell membranes are shed into urine from injured podocytes: a novel phenomenon of podocyte injury. J Am Soc Nephrol 2005;16:408-16. [Crossref] [PubMed]
- Garin EH, Diaz LN, Mu W, et al. Urinary CD80 excretion increases in idiopathic minimal-change disease. J Am Soc Nephrol 2009;20:260-6. [Crossref] [PubMed]
- Ning L, Suleiman HY, Miner JH. Synaptopodin Is Dispensable for Normal Podocyte Homeostasis but Is Protective in the Context of Acute Podocyte Injury. J Am Soc Nephrol 2020;31:2815-32. [Crossref] [PubMed]
- Wang G, Lai FM, Lai KB, et al. Intra-renal and urinary mRNA expression of podocyte-associated molecules for the estimation of glomerular podocyte loss. Ren Fail 2010;32:372-9. [Crossref] [PubMed]
- Wang R, Yao C, Liu F. Association between Renal Podocalyxin Expression and Renal Dysfunction in Patients with Diabetic Nephropathy: A Single-Center, Retrospective Case-Control Study. Biomed Res Int 2020;2020:7350781. [Crossref] [PubMed]
- Kwon SK, Kim SJ, Kim HY. Urine synaptopodin excretion is an important marker of glomerular disease progression. Korean J Intern Med 2016;31:938-43. [Crossref] [PubMed]
- Jim B, Jean-Louis P, Qipo A, et al. Podocyturia as a diagnostic marker for preeclampsia amongst high-risk pregnant patients. J Pregnancy 2012;2012:984630. [Crossref] [PubMed]
- Thomas PE, Wharram BL, Goyal M, et al. GLEPP1, a renal glomerular epithelial cell (podocyte) membrane protein-tyrosine phosphatase. Identification, molecular cloning, and characterization in rabbit. J Biol Chem 1994;269:19953-62. [Crossref] [PubMed]
- Ozaltin F, Ibsirlioglu T, Taskiran EZ, et al. Disruption of PTPRO causes childhood-onset nephrotic syndrome. Am J Hum Genet 2011;89:139-47. [Crossref] [PubMed]
- Jia W, Li H, He YW. The extracellular matrix protein mindin serves as an integrin ligand and is critical for inflammatory cell recruitment. Blood 2005;106:3854-9. [Crossref] [PubMed]
- Murakoshi M, Tanimoto M, Gohda T, et al. Mindin: a novel marker for podocyte injury in diabetic nephropathy. Nephrol Dial Transplant 2011;26:2153-60. [Crossref] [PubMed]
- Kreidberg JA, Donovan MJ, Goldstein SL, et al. Alpha 3 beta 1 integrin has a crucial role in kidney and lung organogenesis. Development 1996;122:3537-47. [Crossref] [PubMed]
- Nicolaou N, Margadant C, Kevelam SH, et al. Gain of glycosylation in integrin α3 causes lung disease and nephrotic syndrome. J Clin Invest 2012;122:4375-87. [Crossref] [PubMed]
- Wei C, El Hindi S, Li J, et al. Circulating urokinase receptor as a cause of focal segmental glomerulosclerosis. Nat Med 2011;17:952-60. [Crossref] [PubMed]
- Hayek SS, Koh KH, Grams ME, et al. A tripartite complex of suPAR, APOL1 risk variants and αvβ3 integrin on podocytes mediates chronic kidney disease. Nat Med 2017;23:945-53. [Crossref] [PubMed]
- Rooney IA, Davies A, Griffiths D, et al. The complement-inhibiting protein, protectin (CD59 antigen), is present and functionally active on glomerular epithelial cells. Clin Exp Immunol 1991;83:251-6. [Crossref] [PubMed]
- Lehto T, Honkanen E, Teppo AM, et al. Urinary excretion of protectin (CD59), complement SC5b-9 and cytokines in membranous glomerulonephritis. Kidney Int 1995;47:1403-11. [Crossref] [PubMed]
- Morrison AA, Viney RL, Saleem MA, et al. New insights into the function of the Wilms tumor suppressor gene WT1 in podocytes. Am J Physiol Renal Physiol 2008;295:F12-7. [Crossref] [PubMed]
- Kubo K, Miyagawa K, Yamamoto R, et al. Detection of WT1 mRNA in urine from patients with kidney diseases. Eur J Clin Invest 1999;29:824-6. [Crossref] [PubMed]
- Lee H, Han KH, Lee SE, et al. Urinary exosomal WT1 in childhood nephrotic syndrome. Pediatr Nephrol 2012;27:317-20. [Crossref] [PubMed]
- Barisoni L, Kriz W, Mundel P, et al. The dysregulated podocyte phenotype: a novel concept in the pathogenesis of collapsing idiopathic focal segmental glomerulosclerosis and HIV-associated nephropathy. J Am Soc Nephrol 1999;10:51-61. [Crossref] [PubMed]
- Espinel CH. The FENa test. Use in the differential diagnosis of acute renal failure. JAMA 1976;236:579-81. [Crossref] [PubMed]
- Zhu H, Wan H, Duan S, et al. Value of monitoring urine ammonia at time of biopsy in patients with lupus nephritis. BMC Nephrol 2020;21:442. [Crossref] [PubMed]
- Bobulescu IA, Maalouf NM, Capolongo G, et al. Renal ammonium excretion after an acute acid load: blunted response in uric acid stone formers but not in patients with type 2 diabetes. Am J Physiol Renal Physiol 2013;305:F1498-503. [Crossref] [PubMed]
- Kalra S. Sodium Glucose Co-Transporter-2 (SGLT2) Inhibitors: A Review of Their Basic and Clinical Pharmacology. Diabetes Ther 2014;5:355-66. [Crossref] [PubMed]
- You R, Zheng H, Xu L, et al. Decreased urinary uromodulin is potentially associated with acute kidney injury: a systematic review and meta-analysis. J Intensive Care 2021;9:70. [Crossref] [PubMed]
- El-Achkar TM, Wu XR, Rauchman M, et al. Tamm-Horsfall protein protects the kidney from ischemic injury by decreasing inflammation and altering TLR4 expression. Am J Physiol Renal Physiol 2008;295:F534-44. [Crossref] [PubMed]
- Matafora V, Cuccurullo M, Beneduci A, et al. Early markers of Fabry disease revealed by proteomics. Mol Biosyst 2015;11:1543-51. [Crossref] [PubMed]
- Tsai CY, Wu TH, Yu CL, et al. Increased excretions of beta2-microglobulin, IL-6, and IL-8 and decreased excretion of Tamm-Horsfall glycoprotein in urine of patients with active lupus nephritis. Nephron 2000;85:207-14. [Crossref] [PubMed]
- Hoyer JR, Resnick JS, Michael AF, et al. Ontogeny of Tamm-Horsfall urinary glycoprotein. Lab Invest 1974;30:757-61. [PubMed]
- Botelho TE, Pereira AK, Teixeira PG, et al. Uromodulin: a new biomarker of fetal renal function? J Bras Nefrol 2016;38:427-34. [Crossref] [PubMed]
- Yu H, Yanagisawa Y, Forbes MA, et al. Alpha-1-microglobulin: an indicator protein for renal tubular function. J Clin Pathol 1983;36:253-9. [Crossref] [PubMed]
- Smart L, Boyd C, Litton E, et al. A randomised controlled trial of succinylated gelatin (4%) fluid on urinary acute kidney injury biomarkers in cardiac surgical patients. Intensive Care Med Exp 2021;9:48. [Crossref] [PubMed]
- Zhang Y, Zhang B, Wang D, et al. Evaluation of Novel Biomarkers for Early Diagnosis of Acute Kidney Injury in Asphyxiated Full-Term Newborns: A Case-Control Study. Med Princ Pract 2020;29:285-91. [Crossref] [PubMed]
- Huart J, Bouquegneau A, Lutteri L, et al. Proteinuria in COVID-19: prevalence, characterization and prognostic role. J Nephrol 2021;34:355-64. [Crossref] [PubMed]
- Ichimura T, Bonventre JV, Bailly V, et al. Kidney injury molecule-1 (KIM-1), a putative epithelial cell adhesion molecule containing a novel immunoglobulin domain, is up-regulated in renal cells after injury. J Biol Chem 1998;273:4135-42. [Crossref] [PubMed]
- Ichimura T, Asseldonk EJ, Humphreys BD, et al. Kidney injury molecule-1 is a phosphatidylserine receptor that confers a phagocytic phenotype on epithelial cells. J Clin Invest 2008;118:1657-68. [Crossref] [PubMed]
- Vaidya VS, Ramirez V, Ichimura T, et al. Urinary kidney injury molecule-1: a sensitive quantitative biomarker for early detection of kidney tubular injury. Am J Physiol Renal Physiol 2006;290:F517-29. [Crossref] [PubMed]
- Vaidya VS, Waikar SS, Ferguson MA, et al. Urinary biomarkers for sensitive and specific detection of acute kidney injury in humans. Clin Transl Sci 2008;1:200-8. [Crossref] [PubMed]
- McWilliam SJ, Antoine DJ, Jorgensen AL, et al. Urinary Biomarkers of Aminoglycoside-Induced Nephrotoxicity in Cystic Fibrosis: Kidney Injury Molecule-1 and Neutrophil Gelatinase-Associated Lipocalin. Sci Rep 2018;8:5094. [Crossref] [PubMed]
- Sabbisetti VS, Waikar SS, Antoine DJ, et al. Blood kidney injury molecule-1 is a biomarker of acute and chronic kidney injury and predicts progression to ESRD in type I diabetes. J Am Soc Nephrol 2014;25:2177-86. [Crossref] [PubMed]
- Vaidya VS, Ford GM, Waikar SS, et al. A rapid urine test for early detection of kidney injury. Kidney Int 2009;76:108-14. [Crossref] [PubMed]
- Yang J, Mori K, Li JY, et al. Iron, lipocalin, and kidney epithelia. Am J Physiol Renal Physiol 2003;285:F9-18. [Crossref] [PubMed]
- Schmidt-Ott KM, Mori K, Kalandadze A, et al. Neutrophil gelatinase-associated lipocalin-mediated iron traffic in kidney epithelia. Curr Opin Nephrol Hypertens 2006;15:442-9. [Crossref] [PubMed]
- Mårtensson J, Bellomo R. The rise and fall of NGAL in acute kidney injury. Blood Purif 2014;37:304-10. [Crossref] [PubMed]
- Ho J, Tangri N, Komenda P, et al. Urinary, Plasma, and Serum Biomarkers' Utility for Predicting Acute Kidney Injury Associated With Cardiac Surgery in Adults: A Meta-analysis. Am J Kidney Dis 2015;66:993-1005. [Crossref] [PubMed]
- Sampaio de Souza Garms D, Cardoso Eid KZ, Burdmann EA, et al. The Role of Urinary Biomarkers as Diagnostic and Prognostic Predictors of Acute Kidney Injury Associated With Vancomycin. Front Pharmacol 2021;12:705636. [Crossref] [PubMed]
- Xu Y, Xie Y, Shao X, et al. L-FABP: A novel biomarker of kidney disease. Clin Chim Acta 2015;445:85-90. [Crossref] [PubMed]
- Sun T, Qu S, Huang T, et al. Rapid and sensitive detection of L-FABP for prediction and diagnosis of acute kidney injury in critically ill patients by chemiluminescent immunoassay. J Clin Lab Anal 2021;35:e24051. [Crossref] [PubMed]
- Sato R, Suzuki Y, Takahashi G, et al. A newly developed kit for the measurement of urinary liver-type fatty acid-binding protein as a biomarker for acute kidney injury in patients with critical care. J Infect Chemother 2015;21:165-9. [Crossref] [PubMed]
- Kashani K, Cheungpasitporn W, Ronco C. Biomarkers of acute kidney injury: the pathway from discovery to clinical adoption. Clin Chem Lab Med 2017;55:1074-89. [Crossref] [PubMed]
- Kashani K, Al-Khafaji A, Ardiles T, et al. Discovery and validation of cell cycle arrest biomarkers in human acute kidney injury. Crit Care 2013;17:R25. [Crossref] [PubMed]
- Melnikov VY, Ecder T, Fantuzzi G, et al. Impaired IL-18 processing protects caspase-1-deficient mice from ischemic acute renal failure. J Clin Invest 2001;107:1145-52. [Crossref] [PubMed]
- Lin X, Yuan J, Zhao Y, et al. Urine interleukin-18 in prediction of acute kidney injury: a systemic review and meta-analysis. J Nephrol 2015;28:7-16. [Crossref] [PubMed]
- Krawczeski CD, Goldstein SL, Woo JG, et al. Temporal relationship and predictive value of urinary acute kidney injury biomarkers after pediatric cardiopulmonary bypass. J Am Coll Cardiol 2011;58:2301-9. [Crossref] [PubMed]
- Hall IE, Yarlagadda SG, Coca SG, et al. IL-18 and urinary NGAL predict dialysis and graft recovery after kidney transplantation. J Am Soc Nephrol 2010;21:189-97. [Crossref] [PubMed]
- Menez S, Ju W, Menon R, et al. Urinary EGF and MCP-1 and risk of CKD after cardiac surgery. JCI Insight 2021;6:147464. [Crossref] [PubMed]
- Satirapoj B, Dispan R, Radinahamed P, et al. Urinary epidermal growth factor, monocyte chemoattractant protein-1 or their ratio as predictors for rapid loss of renal function in type 2 diabetic patients with diabetic kidney disease. BMC Nephrol 2018;19:246. [Crossref] [PubMed]
- Gao L, Zhong X, Jin J, et al. Potential targeted therapy and diagnosis based on novel insight into growth factors, receptors, and downstream effectors in acute kidney injury and acute kidney injury-chronic kidney disease progression. Signal Transduct Target Ther 2020;5:9. [Crossref] [PubMed]
- Toubeau G, Nonclercq D, Zanen J, et al. Renal tissue expression of EGF and EGF receptor after ischaemic tubular injury: an immunohistochemical study. Exp Nephrol 1994;2:229-39. [PubMed]
- Yano T, Yazima S, Hagiwara K, et al. Activation of epidermal growth factor receptor in the early phase after renal ischemia-reperfusion in rat. Nephron 1999;81:230-3. [Crossref] [PubMed]
- Norvik JV, Harskamp LR, Nair V, et al. Urinary excretion of epidermal growth factor and rapid loss of kidney function. Nephrol Dial Transplant 2021;36:1882-92. [Crossref] [PubMed]
- Matsuda A, Jacob A, Wu R, et al. Milk fat globule-EGF factor VIII in sepsis and ischemia-reperfusion injury. Mol Med 2011;17:126-33. [Crossref] [PubMed]
- Kok HM, Falke LL, Goldschmeding R, et al. Targeting CTGF, EGF and PDGF pathways to prevent progression of kidney disease. Nat Rev Nephrol 2014;10:700-11. [Crossref] [PubMed]
- Christov M, Neyra JA, Gupta S, et al. Fibroblast Growth Factor 23 and Klotho in AKI. Semin Nephrol 2019;39:57-75. [Crossref] [PubMed]
- Christov M, Waikar SS, Pereira RC, et al. Plasma FGF23 levels increase rapidly after acute kidney injury. Kidney Int 2013;84:776-85. [Crossref] [PubMed]
- Leaf DE, Jacob KA, Srivastava A, et al. Fibroblast Growth Factor 23 Levels Associate with AKI and Death in Critical Illness. J Am Soc Nephrol 2017;28:1877-85. [Crossref] [PubMed]
- Hoste E, Bihorac A, Al-Khafaji A, et al. Identification and validation of biomarkers of persistent acute kidney injury: the RUBY study. Intensive Care Med 2020;46:943-53. [Crossref] [PubMed]
- Chung AC, Lan HY. Chemokines in renal injury. J Am Soc Nephrol 2011;22:802-9. [Crossref] [PubMed]
- De Loor J, Decruyenaere J, Demeyere K, et al. Urinary chitinase 3-like protein 1 for early diagnosis of acute kidney injury: a prospective cohort study in adult critically ill patients. Crit Care 2016;20:38. [Crossref] [PubMed]
- Maddens B, Ghesquière B, Vanholder R, et al. Chitinase-like proteins are candidate biomarkers for sepsis-induced acute kidney injury. Mol Cell Proteomics 2012;11:M111.013094.
- Hoste EA, Vaara ST, De Loor J, et al. Urinary cell cycle arrest biomarkers and chitinase 3-like protein 1 (CHI3L1) to detect acute kidney injury in the critically ill: a post hoc laboratory analysis on the FINNAKI cohort. Crit Care 2020;24:144. [Crossref] [PubMed]
- Conroy AL, Hawkes MT, Elphinstone R, et al. Chitinase-3-like 1 is a biomarker of acute kidney injury and mortality in paediatric severe malaria. Malar J 2018;17:82. [Crossref] [PubMed]
- Hall IE, Stern EP, Cantley LG, et al. Urine YKL-40 is associated with progressive acute kidney injury or death in hospitalized patients. BMC Nephrol 2014;15:133. [Crossref] [PubMed]
- Wang S, Hu M, Qian Y, et al. CHI3L1 in the pathophysiology and diagnosis of liver diseases. Biomed Pharmacother 2020;131:110680. [Crossref] [PubMed]
- Soylemezoglu O, Wild G, Dalley AJ, et al. Urinary and serum type III collagen: markers of renal fibrosis. Nephrol Dial Transplant 1997;12:1883-9. [Crossref] [PubMed]
- Ghoul BE, Squalli T, Servais A, et al. Urinary procollagen III aminoterminal propeptide (PIIINP): a fibrotest for the nephrologist. Clin J Am Soc Nephrol 2010;5:205-10. [Crossref] [PubMed]
- Ix JH, Biggs ML, Mukamal K, et al. Urine Collagen Fragments and CKD Progression-The Cardiovascular Health Study. J Am Soc Nephrol 2015;26:2494-503. [Crossref] [PubMed]
- Teppo AM, Törnroth T, Honkanen E, et al. Urinary amino-terminal propeptide of type III procollagen (PIIINP) as a marker of interstitial fibrosis in renal transplant recipients. Transplantation 2003;75:2113-9. [Crossref] [PubMed]
Cite this article as: Younes-Ibrahim MS, Younes-Ibrahim M. Biomarkers and kidney diseases: a brief narrative review. J Lab Precis Med 2022;7:20.