Clinical utility of tubular markers in kidney disease: a narrative review
Introduction
Owing to the aging of the population, the number of patients with chronic kidney disease (CKD) is increasing (1). Kidney function gradually declines with advancing age due to deterioration of the nephron, which is the functional unit of the kidney (2,3). Furthermore, reduced kidney function is associated with a high risk for the onset of cardiovascular events (4,5). Consequently, aggressive medical interventions are required following the development of such events. Nevertheless, these interventions occasionally lead to the occurrence of acute kidney injury (AKI) owing to renal ischemia or the use of contrast media (6-8). Severe kidney disease can easily progress to end-stage renal disease (ESRD), requiring renal replacement therapy (i.e., hemodialysis, continuous ambulatory peritoneal dialysis) and renal transplantation. In addition, there is a well-established association between kidney dysfunction and heart failure, which is termed “Cardiorenal syndrome” (9,10). However, currently, there is a limited number of novel treatments against severe kidney disease. Therefore, early diagnosis of kidney disease and identification of patients at high risk of kidney disease progression are warranted for the administration of appropriate treatments at an early phase.
Serum creatinine is widely used as a marker for the evaluation of kidney function. However, change in serum creatinine levels is slow; thereby delaying the early diagnosis and intervention, particularly in AKI (11-13). Therefore, additional useful markers are required in clinical practice. The degree of tubulointerstitial damage is strongly associated with poor renal prognosis versus that of glomerular damage. Hence, the marker which accurately reflects pathophysiological changes in the tubulointerstitium is useful for providing appropriate treatment to the patients with kidney disease. Recently, new urinary tubular markers were established, and their clinical utility was widely investigated in AKI and CKD induced by various causes. This review focuses on newly established urinary tubular markers, namely urinary [tissue inhibitor of metalloproteinases-2 (TIMP-2)]*[insulin-like growth factor binding protein-7 (IGFBP7)] and L-type fatty acid binding protein (L-FABP) were focused (Table 1). We present the following article in accordance with the Narrative Review reporting checklist (available at https://jlpm.amegroups.com/article/view/10.21037/jlpm-22-24/rc).
Table 1
Characteristics | TIMP-2 | IGFBP7 | L-FABP |
---|---|---|---|
Molecule weight | 24 kDa | 29 kDa | 14 kDa |
Expression tubules in human kidney | Distal tubules | Proximal tubules | Proximal tubules |
Related function | Cell cycle arrest | Cell cycle arrest | Fatty metabolism |
Scavenging of reactive oxygen species | |||
Measuring method | NephroCheck | ELISA | |
LTIA | |||
POC assay kit | |||
Clinical utility in kidney disease | AKI | AKI and CKD |
TIMP-2, tissue inhibitor of metalloproteinases-2; IGFBP7, insulin-like growth factor binding protein-7; L-FABP, L-type fatty acid binding protein; ELISA, enzyme-linked immunosorbent assay; LTIA, latex-enhanced immunoturbidimetric assay; POC, point-of-care; AKI, acute kidney injury; CKD, chronic kidney disease.
Methods
A literature search of the electronic databases MEDLINE (January 2014 to February 2022) was conducted using search terms of “urinary [TIMP-2]*[IGFBP7]”, “urinary L-FABP”, “kidney disease”, and “COVID-19”. Original articles, which were written in English and show clinical usefulness of urinary [TIMP-2]*[IGFBP7] or urinary L-FABP, were mainly reviewed.
Discussion
Urinary [TIMP-2]*[IGFBP7] as a marker of AKI
In human kidneys, TIMP-2 is expressed mostly in distal tubules, while IGFBP7 is expressed in proximal tubules (14). Both TIMP-2 and IGFBP7 are related to cell cycle arrest. A recent study indicated that increased glomerular filtration or decreased tubular reabsorption may be the mechanism underlying the increase in urinary [TIMP-2]*[IGFBP7] in AKI (15); moreover, upregulation of TIMP-2 and IGFBP7 in response to tubular stress, leading to increased urinary excretion, has been reported (16).
Urinary [TIMP-2]*[IGFBP7] is useful for the early diagnosis of AKI and prediction of disease severity (17,18). In 2014, the U.S. Food and Drug Administration approved the clinical application of NephroCheck, measuring urinary [TIMP-2]*[IGFBP7], for the diagnosis of AKI. A study in adult patients at the intensive care unit (ICU), analyzing urinary samples collected within 18h of study enrollment, demonstrated that urinary [TIMP-2]*[IGFBP7] predicts the onset of severe AKI, sustained kidney dysfunction, or death within 30 days (18). The proposed cut-off value of urinary [TIMP-2]*[IGFBP7] for AKI was proposed as 0.3 (ng/mL)2/1,000 (19,20). Furthermore, in the patients at higher risk of AKI determined using NephroCheck, treatment for AKI according to the Kidney Disease: Improving Global Outcomes guidelines prior to intervention prevented the onset of cardiac surgery-associated AKI. However, this approach did not improve the survival rate (21). It has been shown that urinary [TIMP-2]*[IGFBP7] for the prediction of AKI is not useful in aortic surgery and orthotopic liver transplantation, apart from cardiac surgery (22,23). Regarding the occurrence of AKI after emergency laparotomy in patients with sepsis, research has revealed that there were no significant differences in urinary [TIMP-2]*[IGFBP7] between the AKI and non-AKI groups (24). At present, the usefulness of urinary [TIMP-2]*[IGFBP7] for the diagnosis of AKI induced by various causes remains to be determined.
Urinary L-FABP as a marker of AKI and CKD
L-FABP, which has a molecular weight of 14 kDa, is expressed in human proximal tubules. L-FABP is not expressed in murine kidneys. Hence, the dynamics of renal L-FABP in various kidney diseases have been assessed in numerous experimental studies using human L-FABP chromosomal transgenic mice because (25). Renal L-FABP expression was consistently upregulated and the urinary excretion of L-FABP was increased in different renal experimental models (25). L-FABP has a renoprotective function by accelerating the fatty metabolism and scavenging of reactive oxygen species (25). In human renal tissues obtained through renal biopsy, the levels of urinary L-FABP were significantly correlated with the degree of tubulointerstitial damage (26,27); therefore, urinary L-FABP is considered to be a tubular marker. The clinical usefulness of urinary L-FABP in various kidney diseases has been demonstrated in studies performed worldwide. Of note, in 2011, the measurement of urinary L-FABP in both AKI and CKD was approved by the Ministry of Health, Labor and Welfare in Japan. We previously reviewed the relationship between urinary L-FABP and kidney disease (28-30). In the present review, we discuss recent evidence regarding urinary L-FABP (Table 2).
Table 2
Disease | Type of clinical study | Situation | Clinical utility | Reference number | Year |
---|---|---|---|---|---|
AKI | Prospective and single center study | Sepsis | Prediction of sepsis severity | (31) | 2017 |
Meta-analysis | Different situations (e.g., ICU, surgery, and injection of contrast media) | Prediction of AKI | (32) | 2022 | |
Prospective and single center study | OSR of an abdominal aortic aneurysm | Prediction of AKI | (33) | 2021 | |
Different cut-off levels of urinary L-FABP for predicting AKI according to the position of AXC | |||||
Prospective and single center study | Emergency laparotomy for acute abdomen by disease of the digestive system | Prediction of AKI | (24) | 2022 | |
In silico study using CPV® | Percutaneous cardiovascular interventions | Prediction of CI-AKI | (34) | 2022 | |
AKI to CKD | Prospective and single center study | OSR of an abdominal aortic aneurysm | Prediction of chronic renal dysfunction after AKI | (33) | 2021 |
Prospective and single center study | Non-surgical cardiac ICUs | Prediction of all-cause mortality or progression to ESRD after AKI | (35) | 2020 | |
CKD | Prospective and multicenter study | CKD with and without type 2 diabetes | Prediction of cardiovascular event and ESRD | (36) | 2016 |
Prospective and single center study | Type 1 diabetes without albuminuria | Prediction of mortality and stroke | (37) | 2017 | |
Prospective and single center study | Hypertension | Prediction of cardiovascular morbidity and mortality, and progression of CKD | (38) | 2020 | |
Aging | Prospective and single center study | Healthy adults without CKD | Possibility of reflecting the degree of age-related nephrosclerosis | (39,40) | 2017, 2018 |
COVID-19 | Prospective and single center study | COVID-19 | Prediction of COVID-19 severity | (41) | 2021 |
Prospective and single center study | COVID-19 | Prediction of COVID-19 severity | (42) | 2020 |
L-FABP, L-type fatty acid binding protein; AKI, acute kidney injury; CKD, chronic kidney disease; COVID-19, coronavirus disease 2019; CPV®, Clinical Performance and Value; ICU, intensive care unit; OSR, open surgical repair; AXC, aortic cross-clamping; CI-AKI, contrast media-induced AKI; ESRD, end-stage renal disease.
Characteristics of urinary L-FABP
Renal hypoxia is a risk factor for the progression of kidney disease (43). Yamamoto et al. investigated the relationship between renal hypoxia and urinary L-FABP. Using an intravital video charge-coupled device, they reported that the urinary levels of L-FABP were significantly decreased following an increase in renal peritubular capillary blood flow in a recipient of a living-related kidney transplant; however, the levels of urinary N-acetyl-β-d-glucosaminidase, α1-microglobulin, and β2-microglobulin were not decreased (44). In addition, we reported that the levels of urinary L-FABP showed a significant negative correlation with the degree of renal peritubular capillary blood flow, measured by diffuse correlation spectroscopy in experimental models of type 2 diabetes (45). Furthermore, renal hypoxia is induced by anemia, which decreases the delivery of oxygen to tissues. Urinary L-FABP levels increased with the progression of anemia in type 2 diabetes (46) and were significantly correlated with the degree of anemia (47). According to these studies, apart from other markers, urinary L-FABP may reflect the degree of renal hypoxia.
Hypoxia-inducible factor-1α (HIF-1α) is upregulated by renal hypoxia in proximal tubules and binds to HIF-1α-binding sites which exist in the promotor region of target genes, leading to the upregulation of those genes (48). As L-FABP has HIF-1α binding sites in its promoter region, renal hypoxia may upregulate the expression of renal L-FABP and accelerate its urinary excretion (49). Therefore, urinary L-FABP may reflect renal microcirculation. From this perspective, urinary L-FABP may be superior to other markers.
Measurements of urinary L-FABP
To measure urinary L-FABP, monoclonal antibodies against human L-FABP were produced and a two-step sandwich enzyme-linked immunosorbent assay (ELISA) was established (50,51). Thereafter, a latex-enhanced immunoturbidimetric assay was developed for the simple, rapid, and highly sensitive (similar to ELISA) measurement of urinary L-FABP (52). Furthermore, a highly sensitive urinary L-FABP kit (39) and a point-of-care (POC) assay kit (31) for the qualitative analysis of urinary L-FABP were recently established. The urinary POC kit offers simple measurements for early diagnosis and intervention.
Urinary L-FABP in AKI
Recently, a meta-analysis was conducted on the clinical utility of urinary (n=25 studies) and plasma (n=2 studies) L-FABP for predicting AKI (32). The estimated sensitivity [0.74, 95% confidence interval (CI): 0.69–0.80)], specificity (0.78, 95% CI: 0.71–0.83), and the area under the receiver operating curve (0.82, 95% CI: 0.79–0.85) of L-FABP suggested that it is a reliable marker with moderate accuracy for predicting AKI in different situations (e.g., ICU, surgery, and injection of contrast media). The cut-off levels of urinary L-FABP for the prediction of AKI varied in clinical studies because the cause of AKI is heterogenous and the invasiveness in each type of surgery differs. In patients undergoing open surgical repair (OSR) of an abdominal aortic aneurysm, the cut-off levels of urinary L-FABP for predicting AKI differed according to the position of aortic cross-clamping (AXC) (33). Urinary L-FABP increased to maximum levels at 2 h after AXC, regardless of the occurrence of AKI. Nonetheless, the levels of urinary L-FABP at 2 h after AXC were significantly greater in patients who underwent suprarenal AXC than in those who underwent infrarenal AXC. This is because the suprarenal AXC induced renal hypoxia more strongly compared with the infrarenal AXC (33). Therefore, the cut-off levels of urinary L-FABP for predicting AKI were significantly higher in patients undergoing suprarenal AXC than in those with infrarenal AXC (33). The evaluation of urinary L-FABP levels in clinical practice should be adapted according to the cause of AKI and type of surgery.
In addition, AKI is a risk factor for CKD and ESRD (53,54), and is related to mortality (55,56). Therefore, the relationship between urinary L-FABP and adverse outcomes in the chronic phase of kidney disease after AKI has been investigated. In patients with onset of AKI who underwent OSR of an abdominal aortic aneurysm, the levels of urinary L-FABP at 2 h after AXC were significantly correlated with reductions in estimated glomerular filtration rate at 3 years after surgery (33). In another study with an observation period of 41 months (n=1,119), involving a heterogenous cohort of patients treated at non-surgical cardiac ICUs, urinary L-FABP on admission was an independent predictor for the primary endpoint, namely all-cause mortality (n=228, approximately 60% cardiovascular deaths) or progression to ESRD (n=17) (35). In addition to creatine-defined AKI, measurement of urinary L-FABP was useful for the identification of patients at high risk for the primary endpoint.
The POC kit of urinary L-FABP may be useful for the early prediction of AKI in clinical practice. A study investigated patients (n=48) who underwent emergency laparotomy for acute abdomen by disease of the digestive system. The qualitative analysis of L-FABP for the prediction of AKI during the perioperative period showed perfect specificity (100%) before the operation and high specificity (>91.9%) at 2, 24, 48, and 72 h after the operation; however, it showed lower sensitivity (24). The L-FABP POC kit may be useful for the discrimination of patients at low risk of AKI. Furthermore, research has demonstrated the clinical usefulness of the L-FABP POC kit for the prediction of contrast media-induced AKI (CI-AKI) in percutaneous cardiovascular interventions (34). CI-AKI is a major cause of AKI in the hospital setting. An in silico study using Clinical Performance and Value (CPV®) vignettes showed that cardiologists were able to correctly identify the patients at high risk of CI-AKI using the L-FABP POC kit (34).
Urinary L-FABP in CKD
Urinary L-FABP is a useful tubular marker for the identification of patients at high risk of CKD progression (28-30). In our previous multicenter trial involving non-diabetic patients with CKD, urinary L-FABP exhibited higher sensitivity for predicting the progression of CKD compared with urinary albumin (51). In addition, these results were corroborated by finding in patients with diabetic nephropathy (DN) (57). DN is a main cause of progression to ESRD. Hence, the detection of the patients at high risk of progression to ESRD is necessary for the administration of effective treatments at an early stage of DN (58). In the stage of normoalbuminuria, urinary L-FABP levels were significantly higher in patients with diabetes versus healthy controls (57,59). Moreover, higher level of urinary L-FABP was an independent risk factor for the progression of DN (28,30).
Furthermore, it is well established that CKD can be easily complicated by the onset of cardiovascular diseases (4). In clinical practice, it is necessary to consider measures for the prevention of cardiovascular events in addition to the management of CKD. Therefore, the relationships between urinary L-FABP and the onset of cardiovascular events in patients with CKD were investigated (36,60). Our multicenter trial with an observation period of approximately 3.8 years (n=244) showed that higher level of urinary L-FABP was an independent risk factor for the primary endpoint, defined as the onset of a cardiovascular event or progression of ESRD [higher urinary L-FABP, hazard ratio (HR): 1.34] (36). A study involving type 1 diabetic patients without albuminuria showed that urinary L-FABP was associated with the onset of stroke (37). Furthermore, a 5-year follow-up study of patients with non-diabetic hypertension (n=197) showed that urinary L-FABP was able to predict cardiovascular morbidity and mortality (higher urinary L-FABP, HR: 1.21), as well as progression of CKD (higher urinary L-FABP, HR: 1.19) (38).
Urinary L-FABP in aging
Healthy aging is associated with changes in both renal macrostructure and microstructure caused by renal arteriosclerosis/arteriolosclerosis, which is defined as fibrous thickening or hyalinosis of the vascular intima (3,61). It is thought that renal arteriosclerosis/arteriolosclerosis is considered to provoke renal ischemia or hypoxia, leading to glomerulosclerosis, tubular atrophy, and interstitial fibrosis. Such renal pathological changes are referred to as nephrosclerosis and are related to a decrease in renal function. In older adults with age-related nephrosclerosis, hypertension or other relevant comorbidities can easily cause kidney disease, thereby increasing the risk of ESRD (3). Therefore, evaluation of the degree of age-related nephrosclerosis and appropriate management strategies against nephrosclerosis may contribute to the prevention of progression to ESRD and prolong the healthy life expectancy in older adults. However, monitoring markers for age-related nephrosclerosis have not been established yet.
Kosaki et al. reported that the levels of urinary L-FABP in healthy adults without CKD increased along with aging, but remained within the normal reference range (39). Furthermore, they showed that urinary L-FABP levels were negatively correlated with physical capacity, evaluated by maximal oxygen consumption and grip strength. It was also demonstrated that habitual aerobic exercise increased physical activity and maximal oxygen consumption, and decreased urinary L-FABP levels (39,40). Considering that urinary L-FABP may indicate renal hypoxia, it may also reflect the degree of the age-related nephrosclerosis and be useful for its monitoring. Furthermore, habitual aerobic exercise may improve renal hemodynamics and prevent the progression of age-related nephrosclerosis.
Urinary L-FABP in coronavirus disease 2019 (COVID-19)
Since 2019, COVID-19 induced by severe acute respiratory syndrome coronavirus type 2 (SARS-CoV-2) has become a public health problem worldwide. SARS-CoV-2 infects human cells via the angiotensin-converting enzyme 2 (ACE2) expressed in different cells, such as alveolar type II cells in the lung, renal proximal tubule cells, cardiomyocytes, epithelial cells of the ileum and esophagus, urothelial cells of the bladder, and vascular endothelial cells (62). Also, it induces systemic inflammation with release of various inflammatory cytokines, leading to a cytokine storm which causes damage to multiple organs (63). In addition, injury of endothelial cells increases the risk of thrombosis, such as cerebral infarction and myocardial infarction (64). Because the degree of organ damage or thrombosis is strongly associated with mortality, early detection of patients at high risk is needed to determine the treatment against COVID-19. A study evaluated the levels of urinary L-FABP in patients with COVID-19 (n=123) using a POC assay kit by 48 h after admission. The levels of urinary L-FABP were significantly higher levels in patients with a primary event (i.e., death, cerebral infarction, myocardial infarction, and stroke) than those without an event (41). Another report (n=49) also indicated that patients with high levels of urinary L-FABP, which were measured using the POC assay kit for 72 h after admission, could easily develop severe disease (42). Urinary L-FABP may be useful for the early determination of COVID-19 patients at high risk of critical illness.
Urinary L-FABP may be useful in various disease because renal hypoxia may be induced in different situation in addition to kidney disease (Table 2).
Conclusions
Tubular markers may reflect various renal and systemic pathological conditions. While urinary [TIMP-2]*[IGFBP7] may be useful for predicting the severity of AKI related to cardiac surgery, urinary L-FABP cloud improve management for patients with kidney diseases, such as AKI, AKI to CKD, and CKD (Figure 1). Furthermore, urinary L-FABP may predict the progression of age-related nephrosclerosis and COVID-19. Early utilization of an accurate marker may provide beneficial effects on the prognosis of kidney disease and patient survival.
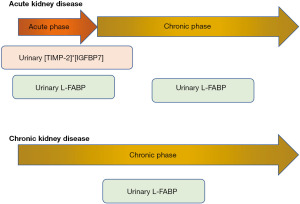
Acknowledgments
Funding: None.
Footnote
Provenance and Peer Review: This article was commissioned by the Guest Editor (Tatsuo Shimosawa) for the series “New Biomarkers in Non-communicable Diseases” published in Journal of Laboratory and Precision Medicine. The article has undergone external peer review.
Reporting Checklist: The authors have completed the Narrative Review reporting checklist. Available at https://jlpm.amegroups.com/article/view/10.21037/jlpm-22-24/rc
Conflicts of Interest: Both authors have completed the ICMJE uniform disclosure form (available at https://jlpm.amegroups.com/article/view/10.21037/jlpm-22-24/coif). The series “New Biomarkers in Non-communicable Diseases” was commissioned by the editorial office without any funding or sponsorship. The authors have no conflicts of interest to declare.
Ethical Statement: The authors are accountable for all aspects of the work in ensuring that questions related to the accuracy or integrity of any part of the work are appropriately investigated and resolved.
Open Access Statement: This is an Open Access article distributed in accordance with the Creative Commons Attribution-NonCommercial-NoDerivs 4.0 International License (CC BY-NC-ND 4.0), which permits the non-commercial replication and distribution of the article with the strict proviso that no changes or edits are made and the original work is properly cited (including links to both the formal publication through the relevant DOI and the license). See: https://creativecommons.org/licenses/by-nc-nd/4.0/.
References
- Centers for Disease Control and Prevention. Chronic Kidney Disease (CKD) Surveillance System. Available online: https://nccd.cdc.gov/CKD/ (Accessed 2/19/2021).
- Denic A, Glassock RJ, Rule AD. Structural and Functional Changes With the Aging Kidney. Adv Chronic Kidney Dis 2016;23:19-28. [Crossref] [PubMed]
- Hommos MS, Glassock RJ, Rule AD. Structural and Functional Changes in Human Kidneys with Healthy Aging. J Am Soc Nephrol 2017;28:2838-44. [Crossref] [PubMed]
- Chronic Kidney Disease Prognosis Consortium. Association of estimated glomerular filtration rate and albuminuria with all-cause and cardiovascular mortality in general population cohorts: a collaborative meta-analysis. Lancet 2010;375:2073-81. [Crossref] [PubMed]
- Vallianou NG, Mitesh S, Gkogkou A, et al. Chronic Kidney Disease and Cardiovascular Disease: Is there Any Relationship? Curr Cardiol Rev 2019;15:55-63. [Crossref] [PubMed]
- Djordjević A, Šušak S, Velicki L, et al. Acute kidney injury after open-heart surgery procedures. Acta Clin Croat 2021;60:120-6. [Crossref] [PubMed]
- Lameire N, Kellum JAKDIGO AKI Guideline Work Group. Contrast-induced acute kidney injury and renal support for acute kidney injury: a KDIGO summary (Part 2). Crit Care 2013;17:205. [Crossref] [PubMed]
- Ortega-Loubon C, Fernández-Molina M, Carrascal-Hinojal Y, et al. Cardiac surgery-associated acute kidney injury. Ann Card Anaesth 2016;19:687-98. [Crossref] [PubMed]
- Ronco C, Haapio M, House AA, et al. Cardiorenal syndrome. J Am Coll Cardiol 2008;52:1527-39. [Crossref] [PubMed]
- Ronco C, McCullough PA, Anker SD, et al. Cardiorenal syndromes: an executive summary from the consensus conference of the Acute Dialysis Quality Initiative (ADQI). Contrib Nephrol 2010;165:54-67. [Crossref] [PubMed]
- Malhotra R, Siew ED. Biomarkers for the Early Detection and Prognosis of Acute Kidney Injury. Clin J Am Soc Nephrol 2017;12:149-73. [Crossref] [PubMed]
- Singh R, Dodkins J, Doyle JF, et al. Acute Kidney Injury Biomarkers: What Do They Tell Us? Contrib Nephrol 2018;193:21-34. [Crossref] [PubMed]
- Waikar SS, Bonventre JV. Creatinine kinetics and the definition of acute kidney injury. J Am Soc Nephrol 2009;20:672-9. [Crossref] [PubMed]
- Emlet DR, Pastor-Soler N, Marciszyn A, et al. Insulin-like growth factor binding protein 7 and tissue inhibitor of metalloproteinases-2: differential expression and secretion in human kidney tubule cells. Am J Physiol Renal Physiol 2017;312:F284-96. [Crossref] [PubMed]
- Johnson ACM, Zager RA. Mechanisms Underlying Increased TIMP2 and IGFBP7 Urinary Excretion in Experimental AKI. J Am Soc Nephrol 2018;29:2157-67. [Crossref] [PubMed]
- Ortega LM, Heung M. The use of cell cycle arrest biomarkers in the early detection of acute kidney injury. Is this the new renal troponin? Nefrologia 2018;38:361-7. (Engl Ed). [Crossref] [PubMed]
- Fan W, Ankawi G, Zhang J, et al. Current understanding and future directions in the application of TIMP-2 and IGFBP7 in AKI clinical practice. Clin Chem Lab Med 2019;57:567-76. [Crossref] [PubMed]
- Kashani K, Al-Khafaji A, Ardiles T, et al. Discovery and validation of cell cycle arrest biomarkers in human acute kidney injury. Crit Care 2013;17:R25. [Crossref] [PubMed]
- Bihorac A, Chawla LS, Shaw AD, et al. Validation of cell-cycle arrest biomarkers for acute kidney injury using clinical adjudication. Am J Respir Crit Care Med 2014;189:932-9. [Crossref] [PubMed]
- Hoste EA, McCullough PA, Kashani K, et al. Derivation and validation of cutoffs for clinical use of cell cycle arrest biomarkers. Nephrol Dial Transplant 2014;29:2054-61. [Crossref] [PubMed]
- Meersch M, Schmidt C, Hoffmeier A, et al. Prevention of cardiac surgery-associated AKI by implementing the KDIGO guidelines in high risk patients identified by biomarkers: the PrevAKI randomized controlled trial. Intensive Care Med 2017;43:1551-61. [Crossref] [PubMed]
- Schiefer J, Lichtenegger P, Berlakovich GA, et al. Urinary [TIMP-2]×[IGFBP-7] for predicting acute kidney injury in patients undergoing orthotopic liver transplantation. BMC Nephrol 2019;20:269. [Crossref] [PubMed]
- Waskowski J, Pfortmueller CA, Schenk N, et al. (TIMP2) x (IGFBP7) as early renal biomarker for the prediction of acute kidney injury in aortic surgery (TIGER). A single center observational study. PLoS One 2021;16:e0244658. [Crossref] [PubMed]
- Okuda H, Obata Y, Kamijo-Ikemori A, et al. Quantitative and qualitative analyses of urinary L-FABP for predicting acute kidney injury after emergency laparotomy. J Anesth 2022;36:38-45. [Crossref] [PubMed]
- Kamijo-Ikemori A, Sugaya T, Matsui K, et al. Roles of human liver type fatty acid binding protein in kidney disease clarified using hL-FABP chromosomal transgenic mice. Nephrology (Carlton) 2011;16:539-44. [Crossref] [PubMed]
- Kamijo A, Sugaya T, Hikawa A, et al. Urinary excretion of fatty acid-binding protein reflects stress overload on the proximal tubules. Am J Pathol 2004;165:1243-55. [Crossref] [PubMed]
- Sasaki H, Kamijo-Ikemori A, Sugaya T, et al. Urinary fatty acids and liver-type fatty acid binding protein in diabetic nephropathy. Nephron Clin Pract 2009;112:c148-56. [Crossref] [PubMed]
- Kamijo-Ikemori A, Sugaya T, Ichikawa D, et al. Urinary liver type fatty acid binding protein in diabetic nephropathy. Clin Chim Acta 2013;424:104-8. [Crossref] [PubMed]
- Kamijo-Ikemori A, Sugaya T, Kimura K. Urinary fatty acid binding protein in renal disease. Clin Chim Acta 2006;374:1-7. [Crossref] [PubMed]
- Kamijo-Ikemori A, Sugaya T, Kimura K. Novel urinary biomarkers in early diabetic kidney disease. Curr Diab Rep 2014;14:513. [Crossref] [PubMed]
- Sato E, Kamijo-Ikemori A, Oikawa T, et al. Urinary excretion of liver-type fatty acid-binding protein reflects the severity of sepsis. Renal Replacement Ther 2017;3:26. [Crossref]
- Chiang TH, Yo CH, Lee GH, et al. Accuracy of Liver-Type Fatty Acid-Binding Protein in Predicting Acute Kidney Injury: A Meta-Analysis. J Appl Lab Med 2022;7:421-36. [Crossref] [PubMed]
- Obata Y, Kamijo-Ikemori A, Inoue S. Clinical Utility of Urinary Biomarkers for Prediction of Acute Kidney Injury and Chronic Renal Dysfunction After Open Abdominal Aortic Aneurysm Repair. Int J Nephrol Renovasc Dis 2021;14:371-84. [Crossref] [PubMed]
- Peabody J, Paculdo D, Valdenor C, et al. Clinical Utility of a Biomarker to Detect Contrast-Induced Acute Kidney Injury during Percutaneous Cardiovascular Procedures. Cardiorenal Med 2022;12:11-9. [Crossref] [PubMed]
- Naruse H, Ishii J, Takahashi H, et al. Urinary Liver-Type Fatty-Acid-Binding Protein Predicts Long-Term Adverse Outcomes in Medical Cardiac Intensive Care Units. J Clin Med 2020;9:482. [Crossref] [PubMed]
- Matsui K, Kamijo-Ikemori A, Imai N, et al. Clinical significance of urinary liver-type fatty acid-binding protein as a predictor of ESRD and CVD in patients with CKD. Clin Exp Nephrol 2016;20:195-203. [Crossref] [PubMed]
- Panduru NM, Forsblom C, Saraheimo M, et al. Urinary liver-type fatty acid binding protein is an independent predictor of stroke and mortality in individuals with type 1 diabetes. Diabetologia 2017;60:1782-90. [Crossref] [PubMed]
- Okubo Y, Nakano Y, Tokuyama T, et al. Increased Urinary Liver-Type Fatty Acid-Binding Protein Level Predicts Major Adverse Cardiovascular Events in Patients With Hypertension. Am J Hypertens 2020;33:604-9. [Crossref] [PubMed]
- Kosaki K, Kamijo-Ikemori A, Sugaya T, et al. Relationship between exercise capacity and urinary liver-type fatty acid-binding protein in middle-aged and older individuals. Clin Exp Nephrol 2017;21:810-7. [Crossref] [PubMed]
- Kosaki K, Kamijo-Ikemori A, Sugaya T, et al. Effect of habitual exercise on urinary liver-type fatty acid-binding protein levels in middle-aged and older adults. Scand J Med Sci Sports 2018;28:152-60. [Crossref] [PubMed]
- Tantry US, Bliden KP, Cho A, et al. First Experience Addressing the Prognostic Utility of Novel Urinary Biomarkers in Patients With COVID-19. Open Forum Infect Dis 2021;8:ofab274.
- Katagiri D, Ishikane M, Asai Y, et al. Evaluation of Coronavirus Disease 2019 Severity Using Urine Biomarkers. Crit Care Explor 2020;2:e0170. [Crossref] [PubMed]
- Mimura I, Nangaku M. The suffocating kidney: tubulointerstitial hypoxia in end-stage renal disease. Nat Rev Nephrol 2010;6:667-78. [Crossref] [PubMed]
- Yamamoto T, Noiri E, Ono Y, et al. Renal L-type fatty acid--binding protein in acute ischemic injury. J Am Soc Nephrol 2007;18:2894-902. [Crossref] [PubMed]
- Tanabe J, Ogura Y, Nakabayashi M, et al. The Possibility of Urinary Liver-Type Fatty Acid-Binding Protein as a Biomarker of Renal Hypoxia in Spontaneously Diabetic Torii Fatty Rats. Kidney Blood Press Res 2019;44:1476-92. [Crossref] [PubMed]
- von Eynatten M, Baumann M, Heemann U, et al. Urinary L-FABP and anaemia: distinct roles of urinary markers in type 2 diabetes. Eur J Clin Invest 2010;40:95-102. [Crossref] [PubMed]
- Imai N, Yasuda T, Kamijo-Ikemori A, et al. Distinct roles of urinary liver-type fatty acid-binding protein in non-diabetic patients with anemia. PLoS One 2015;10:e0126990. [Crossref] [PubMed]
- Nangaku M, Inagi R, Miyata T, et al. Hypoxia and hypoxia-inducible factor in renal disease. Nephron Exp Nephrol 2008;110:e1-7. [Crossref] [PubMed]
- Noiri E, Doi K, Negishi K, et al. Urinary fatty acid-binding protein 1: an early predictive biomarker of kidney injury. Am J Physiol Renal Physiol 2009;296:F669-79. [Crossref] [PubMed]
- Kamijo A, Kimura K, Sugaya T, et al. Urinary fatty acid-binding protein as a new clinical marker of the progression of chronic renal disease. J Lab Clin Med 2004;143:23-30. [Crossref] [PubMed]
- Kamijo A, Sugaya T, Hikawa A, et al. Clinical evaluation of urinary excretion of liver-type fatty acid-binding protein as a marker for the monitoring of chronic kidney disease: a multicenter trial. J Lab Clin Med 2005;145:125-33. [Crossref] [PubMed]
- Kamijo-Ikemori A, Sugaya T, Yoshida M, et al. Clinical utility of urinary liver-type fatty acid binding protein measured by latex-enhanced turbidimetric immunoassay in chronic kidney disease. Clin Chem Lab Med 2016;54:1645-54. [Crossref] [PubMed]
- Chawla LS, Bellomo R, Bihorac A, et al. Acute kidney disease and renal recovery: consensus report of the Acute Disease Quality Initiative (ADQI) 16 Workgroup. Nat Rev Nephrol 2017;13:241-57. [Crossref] [PubMed]
- Coca SG, Singanamala S, Parikh CR. Chronic kidney disease after acute kidney injury: a systematic review and meta-analysis. Kidney Int 2012;81:442-8. [Crossref] [PubMed]
- Hoste EAJ, Kellum JA, Selby NM, et al. Global epidemiology and outcomes of acute kidney injury. Nat Rev Nephrol 2018;14:607-25. [Crossref] [PubMed]
- Lewington AJ, Cerdá J, Mehta RL. Raising awareness of acute kidney injury: a global perspective of a silent killer. Kidney Int 2013;84:457-67. [Crossref] [PubMed]
- Kamijo-Ikemori A, Sugaya T, Yasuda T, et al. Clinical significance of urinary liver-type fatty acid-binding protein in diabetic nephropathy of type 2 diabetic patients. Diabetes Care 2011;34:691-6. [Crossref] [PubMed]
- Martínez-Castelao A, Navarro-González JF, Górriz JL, et al. The Concept and the Epidemiology of Diabetic Nephropathy Have Changed in Recent Years. J Clin Med 2015;4:1207-16. [Crossref] [PubMed]
- Nielsen SE, Sugaya T, Tarnow L, et al. Tubular and glomerular injury in diabetes and the impact of ACE inhibition. Diabetes Care 2009;32:1684-8. [Crossref] [PubMed]
- Araki S, Haneda M, Koya D, et al. Predictive effects of urinary liver-type fatty acid-binding protein for deteriorating renal function and incidence of cardiovascular disease in type 2 diabetic patients without advanced nephropathy. Diabetes Care 2013;36:1248-53. [Crossref] [PubMed]
- O'Sullivan ED, Hughes J, Ferenbach DA. Renal Aging: Causes and Consequences. J Am Soc Nephrol 2017;28:407-20. [Crossref] [PubMed]
- Angeli F, Zappa M, Reboldi G, et al. The pivotal link between ACE2 deficiency and SARS-CoV-2 infection: One year later. Eur J Intern Med 2021;93:28-34. [Crossref] [PubMed]
- Moore JB, June CH. Cytokine release syndrome in severe COVID-19. Science 2020;368:473-4. [Crossref] [PubMed]
- Ackermann M, Verleden SE, Kuehnel M, et al. Pulmonary Vascular Endothelialitis, Thrombosis, and Angiogenesis in Covid-19. N Engl J Med 2020;383:120-8. [Crossref] [PubMed]
Cite this article as: Kamijo-Ikemori A, Kimura K. Clinical utility of tubular markers in kidney disease: a narrative review. J Lab Precis Med 2022;7:27.