Investigative algorithms for disorders affecting acidosis: a narrative review
Introduction
Acid balance in the body is tightly controlled with respiratory and renal systems, both of which are essential for acid-base homeostasis. Acute and chronic compensatory mechanisms exist to help maintain the steady internal environment; the respiratory rate is rapidly alterable for acute compensation of metabolic acidosis, and renal compensation provides a more chronic correction of respiratory acidosis (1). The following article will discuss acidosis, respiratory and metabolic, specifically on the laboratory approach to diagnosis.
It should be noted that symptoms caused by changes in pH are not well established as they rarely occur in isolation, e.g., are associated with changes in partial pressure of carbon dioxide (pCO2). This article is not designed to be a comprehensive discussion of the topic, but to present enough information so that the diagnostic schema can be used, and the limitations of the laboratory tests appreciated. As the healthcare team expands the aim of the article is to support clinicians as experience is gained, or who have less exposure in their regular roles to formulating differential diagnoses, and is not meant to be a substitute for thorough clinical assessment. We present the following article in accordance with the Narrative Review reporting checklist (available at https://jlpm.amegroups.com/article/view/10.21037/jlpm-22-9/rc).
Methods
Medline, Google Scholar, OMIM and seminal texts were searched to inform the narrative literature review. Information obtained was used to derive the diagnostic algorithms. The literature was searched over the period September 2021 to January 2022, English language only. For further information please see supplementary information (Table S1).
Metabolism
Acidosis is defined as an arterial blood pH of <7.35, however this may depend on local method and age of patient. Acid is both ingested and produced by metabolism (Figure 1). The metabolic conversion of CO2 to carbonic acid and ultimately hydrogen and bicarbonate ions, catalysed by carbonic anhydrase (shown below), is the basic chemical step responsible for the acid-base state of circulating plasma (and in the kidney) (2).
Knowing the pCO2 is imperative to determine the cause of an acid-base imbalance. An estimate of the length of illness can also help as you may see signs of compensation developing (1).
Compensation
Compensation in the acute phase consists of increased ventilation to ‘blow off’ acid in the form of CO2. As can be seen in the above equation, due to Le Chatelier’s principle, reducing pCO2 will ultimately reduce H+ (3). In chronic acidosis, the kidneys can regenerate more bicarbonate ions to absorb into the circulation as, normally, the kidneys reabsorb all bicarbonate and excrete none (Figure 2). These changes in pCO2 and bicarbonate concentration can be estimated, particularly if the time of onset and type of acidosis is known (Table 1) (1). It is important to note that over-compensation can never occur, and the compensation formulae were established originally in canine models (4). Most of these have been replaced by formulae derived from small human studies. However, although linear, there is scatter and therefore these estimates are approximate only (Table 1) (1).
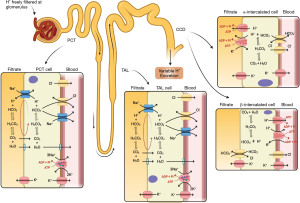
Table 1
Type of acidosis | Change |
---|---|
Rise in [HCO3−] per increase in PaCO2 | |
Acute respiratory acidosis | 0.1 mmol/L per mmHg (0.133 kPa) |
Chronic respiratory acidosis | 0.35 mmol/L per mmHg (0.133 kPa) |
Fall in PaCO2 per decrease in [HCO3−] | |
Metabolic acidosis | 1.2 mmHg (0.160 kPa) per mmol/L |
[K+]p change for every 0.1 reduction in pH | |
Respiratory acidosis | 0.1 mmol/L increase |
Mineral acid metabolic acidosis, e.g., HCL, an inorganic acid with impermeant anion | 0.7 mmol/L increase |
Organic acid metabolic acidosis, e.g., lactic acidosis, permeant anion | No change |
Acute meaning within hours and chronic several days. [HCO3−], concentration of bicarbonate; PaCO2, arterial partial pressure of carbon dioxide; [K+]p, potassium concentration in plasma; HCL, hydrochloric acid.
Hyperkalaemia
Acidosis, particularly in the presence of an impermeable anion, affects potassium concentration causing hyperkalaemia (Table 1) (5). This is due to H+ exchange with intracellular K+, renal sodium pump inhibition, and inhibition of the apical tubular potassium permeability (the latter two reducing K+ excretion with H+ excreted in its stead).
Hypochloraemia and hyperchloraemia
Renal compensation, i.e., bicarbonate generation, requires anion exchange in the kidneys and tissues in order to maintain electrical neutrality. Bicarbonate is exchanged with chloride ions, which is why acidosis is associated with hypochloraemic hyperkalaemia (5). However, hyperchloraemic hypokalaemic acidosis can occur, caused by conditions such as: renal tubular acidosis (RTA), acetazolamide usage, ureteric diversion, and severe diarrhoea, particularly in children (see chloride article in this series of articles). Mechanisms causing hyperchloraemic hypokalaemic acidosis include; metabolism of amino acids and ammonium chloride salts by the liver, volume depletion (hence replacement of the lost sodium bicarbonate with sodium chloride by the kidney), and loss of organic acid anions (6).
Preanalytical considerations of acid base assessment
It is also important to know that pre-analytical issues are common in assessment of acid-base disorders and, although rarer, analytical issues can also occur. The patient must be breathing at their basal rate when the sample is taken, therefore they should be prone and as relaxed as possible (7,8). Specimen tubes should be glass, yet are mostly plastic, which is more practical, but is slightly permeable to oxygen. All air should be expelled from syringes first; under-filling the specimen tube (residual air) will mimic a metabolic acidosis and raise the anion gap (9). Only heparin should be used as an anticoagulant because citrate, EDTA and fluoride alter blood pH, mimicking a high anion gap metabolic acidosis (10,11). Under-filling specimen tubes which contain liquid anticoagulant can lead to dilution artefact, reducing pCO2 and bicarbonate concentration (12). Heparin can be acidic, consequently manufacturing variation may result in spurious acidosis (13). Specimens should be analysed immediately, to prevent metabolism in vitro affecting results (12).
If venous blood is measured instead of arterial, then there will be differences in the respiratory gasses which should be anticipated (low pO2, high pCO2) (14). The issue is that the expected differences may apply to well, stable patients but not to severely ill patients (6). However, adjustment formulae have been validated in an attempt to limit arterial venesection, which is not without risk, and performance may be acceptable as long as the limitations are understood (15). Capillary sampling is also not recommended, as samples can be affected by changes to the peripheral circulation. However, to mitigate this, sites such as the ear lobe that have been ‘arterialised’ (warmed and rubbed to create hyperaemia) can be used. This is not an appropriate method for pO2, and both pH and pCO2 can be affected by sampling technique. In the following paper, we will refer to measurements performed on arterial samples for blood gasses (or serum for other analytes).
Blood gas analysers measure pH, pCO2, and pO2; then calculate the rest of the derivate parameters. These values are all also adjusted for the patient’s temperature, as all these parameters are affected by temperature. This practice, albeit controversial, will affect results depending on whether users correctly enter the temperature on analysis (12). It is however true that the locally reported ‘reference intervals’ apply, irrespective of the patient’s temperature. The alveolar-arterial gradient must use temperature adjusted values to avoid spurious results (16). Direct electrodes remain the dominant method for pH and gas partial pressure measurement therefore reference intervals should be similar between analyser, hospital and country. It is worth noting that ranges not only depend on sample type, e.g., arterial versus venous, but also on patient age, e.g., neonatal and pregnancy differences.
Algorithms for investigating acidosis
An algorithm with suggestions of how to approach the diagnosis of acidosis is provided (Figure 3A,3B). There is also an algorithm for considering a low bicarbonate in isolation (Figure 4A,4B, for a high bicarbonate, please see the alkalosis article in this series). Spuriously low bicarbonate on certain methods has also been reported with no identifiable cause (17). Not all patients will have access to blood gas analysis, particularly ambulant outpatients, and therefore we have included a short algorithm on an isolated abnormal bicarbonate, e.g., from a serum sample. As the acid-base status is not known in these cases, a low bicarbonate is effectively either a metabolic acidosis or compensation for respiratory alkalosis (Figure 4A,4B). Please bear in mind that the tests for an acid-base disorder are never completely diagnostic, and that the conclusion must be weighed by the clinical evidence (1). Pre-existing acid base disorders, mixed disorders, renal impairment, fluid status, and other therapies, will all affect the results; so correlate closely with the clinical situation (9).
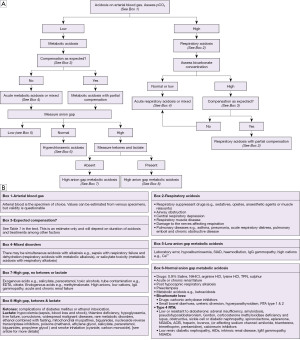
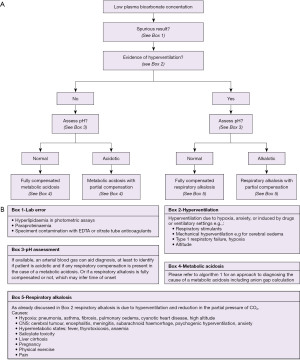
Respiratory acidosis
Respiratory acidosis is caused by a reduction in pulmonary function, hence raised pCO2. This increases the proton concentration, as shown below, where 24 is the equilibrium constant k:
Respiratory acidosis is therefore indicated on an arterial blood gas with low arterial pH and raised pCO2 (<7.35 and >6.0 kPa respectively or as per local ranges). The bicarbonate concentration may be raised if there has been an opportunity to compensate (or there is a coexistent metabolic alkalosis). Venous bloods may demonstrate, in addition, hypochloraemia and hyperkalaemia.
Due to the possibility of mixed pathology, as well as the effects of acute and chronic compensation, one can attempt to estimate the compensation expected (Table 1). Rapid cell buffering (only limited intracellular buffering capability by haemoglobin and proteins), and bicarbonate buffering, occurs in the acute phase (with a rise in bicarbonate concentration), but this response is limited. Longer term (3–5 days), the kidneys compensate by increasing the excretion of protons. The more chronic the compensation, the more likely the pH will be close to normal (if not normal), with further elevations of bicarbonate concentration. To assess whether compensation has taken place as expected, the anticipated change in bicarbonate concentration can be calculated (Table 1). However, the expected change in bicarbonate will depend on whether it is acute, or chronic, due to the underlying differences in mechanism; which can further complicate the utility of these estimates (Table 1).
Chronic compensation of respiratory acidosis is extremely efficient. Therefore, to have a respiratory acidosis, the cause must be acute, or the pre-existing condition has acutely deteriorated (1).
Metabolic acidosis
Metabolic acidosis can be caused by excess acid (either ingestion or production), an inability to excrete acid, or excretion of too much alkali, e.g., drugs and poisons, renal, and gastrointestinal problems. It is diagnosed by demonstrating, on an arterial blood gas, decreased pH, bicarbonate, and pCO2. To compensate, the body will increase the respiratory drive; to blow off CO2 (down to a minimum of approximately 1.3–2.0 kPa, 10–15 mmHg). Compensation starts within 30 minutes, but full compensation takes up to 24 hours. Again, the co-existence of a mixed disorder can be gauged by estimation of the expected changes (Table 1). However, it is important to bear in mind that the minimum pCO2 are only estimates, that anoxic brain injury (brain stem compression, etc.) can reduce ventilation rate (as can some metabolic poisons and opiate overdose), and that co-existent lung disease or respiratory fatigue (e.g., asthma attacks) may be present, all of which can reduce the compensation capability. Once a metabolic acidosis is detected, then calculation of the anion gap can help identify the source of the acid:
Due to the law of electroneutrality, negative and positive charge must be balanced (2). Approximately 95% of all the cations are represented by sodium and potassium, and 85% of all anions by chloride and bicarbonate; therefore, a small gap is expected e.g., 6–10 mmol/L (or 10–14 mmol/L) (2). It is important to note that the anion gap was first calculated using methods such as flame photometry, and that now assays have changed (e.g., automated enzymatic), the anion gap is lower than the initial estimates. Therefore, it is important to validate a local range based on the methods of analysis used (a bicarbonate concentration from the blood gas report will be different from the laboratory due to methodological and specimen differences, e.g., venous versus arterial), especially if variations of the formula above are also employed (2,18). Effectively, the gap consists of ions not routinely measured such as:
In metabolic acidosis, a decrease in serum bicarbonate should cause an equal increase in the serum anion gap (6). If the anion gap is normal, then it would suggest that a hyperchloraemic acidosis is present; with low serum bicarbonate and high serum chloride concentration (Table 2). A high anion gap suggests that there is an unmeasured anion circulating, e.g., an organic acid such as lactate or ketones, or a decrease in cations (potassium or calcium) (Table 2). It should be noted that albumin correlates with anion gap, i.e., as albumin drops so does the gap, by approximately 2.3–2.5 mmol/L per 10 g/L albumin (Table 2) (2).
Table 2
Low anion gap, e.g., <10 mmol/L* |
Consider laboratory error, e.g., overestimated Cl− (bromide or iodine toxicity) or HCO3− (e.g., delayed separation); pseudo-hyponatraemia |
Hypoalbuminaemia, reduction of unmeasured anions; haemodilution particularly SIAD |
High concentration of cations, e.g., Ca2+, Li+ |
Monoclonal, e.g., IgG multiple myeloma, or polyclonal gammopathy |
Normal anion gap, e.g., 10–14 mmol/L |
Ingestion of NH4Cl, arginine or lysine chloride, HCL, sulphur toxicity. Parenteral nutrition |
Acetazolamide if potassium is low |
Acute or chronic renal failure if potassium high or normal; overproduction acidosis, e.g., ketoacidosis** |
Mineralocorticoid deficiency if potassium is high or normal; renal tubular acidosis type 4 |
Diarrhoea or gastrointestinal fistula, ureteric diversion or renal tubular acidosis types 1 and 2 if potassium is low |
High anion gap, e.g., >14 mmol/L (>30 mmol/L is particularly indicative of acid presence) |
Ingestion of salicylate, paracetamol, ethanol, methanol, ethylene glycol |
In born error of metabolism, e.g., organic acidaemias (metabolic alkalosis: hyperalbuminaemia and increased anionic tendency in high pH) |
Increased anions, e.g., PO43−, IgA paraproteins; reduction of cations, e.g., Mg2+, Ca2+; hyperalbuminaemia (dehydration); acute and chronic renal failure |
If ketones are raised consider ketoacidosis from either diabetes mellitus or ethanol intoxication, rarely in born errors of metabolism |
Lactic acidosis: hypovolemia (sepsis, blood loss and shock), thiamine deficiency, hypoglycaemia, drugs1, liver failure, convulsions, widespread malignant diseases, rare metabolic disorders2 |
*, if anion gap is low, consider these causes and then causes of the normal anion gap to help distinguish the aetiology of the acidotic state; **, in overproduction acidosis, the anion gap can be normal depending on filtered load of acid and limited ability of the kidneys to make enough bicarbonate to balance anion excretion. If overwhelmed chloride is exchanged instead resulting in a normal anion gap despite significant acid production, e.g., ketones. 1, Biguanides, e.g., metformin, salicylate, ethanol, methanol, ethylene glycol, high dose fructose and xylitol; 2, type 1 glycogen storage disease (e.g., glucose-6-phosphatase deficiency or von Gierke), pyruvate dehyrogenase/carboxylate deficiency, fructose-1,6-diphosphate deficiency, methylmalonic organic acidosis, Leigh syndrome and oxidative phosphorylation defects. SIAD, syndrome of inappropriate antidiuresis; IgG, immunoglobulin G; HCL, hydrochloric acid; IgA, immunoglobulin A.
The presence of an acid is more likely to be identified if the anion gap is >30 mmol/L, in which case the commonest causes are lactate and ketones (2,19). The gap can be due to changes in ionic status of proteins, phosphate, potassium, and calcium; making gap calculation unreliable. Hence, the more abnormal the anion gap is, the more reliable acid detection is (10). Reduction in the concentrations of the anions, as well as an increased concentration of cations, or the presence of polyclonal or monoclonal immunoglobulin G (IgG) gammaglobinaemia, can make the gap spuriously normal (2). IgG is cationic with immunoglobulin A tending to be anionic, hence having an opposite effect on the anion gap (Table 2). There is wide inter-individual variation in the anion gap. Therefore, a significant change can occur in some individuals, yet they remain within the ‘reference interval’. The time post onset will also affect the anion gap. Toxic alcohols will initially create an osmolar gap, and later an anion gap (20). The therapy instigated, e.g., fluids, can further complicate interpretation (2).
Lactic acid metabolic acidosis
Lactic acid is a strong acid (pKa 3.8) and completely dissociates at physiological pH; with equimolar production of protons for each lactate molecule. Pyruvate, the precursor of lactate, is rarely important as it is usually at a much lower concentration than lactate but it is a strong acid, and dissociates 20 times more than lactate (21). The liver metabolises lactate, and can metabolise it several fold faster than it can be produced in certain tissues, e.g., by skeletal muscle after intense muscular activity (12). Lactate increases in anaerobic respiration. There are two types of lactic acidosis—A, where there is the classic common hypovolaemic/hypoxic unwell patient caused by poor delivery of oxygen to tissues versus B, where there is a poison or metabolic error that is affecting mitochondrial and aerobic respiration (see rare causes section below).
RTA
RTA (Table 3, Figure 5A,5B) occurs when there is a problem with renal handling of either H+ or HCO3−, limiting urinary acidification, resulting in a normal anion gap (hyperchloraemic) metabolic acidosis. There are three main types, 1, 2 and 4, with more details provided in Table 3 (22-24). Type 3 RTA is extremely rare and is caused by mutations resulting in carbonic anhydrase II deficiency, and shares features of both type 1 and type 2 RTA. All are causes of normal anion gap (hyperchloraemic) metabolic acidosis.
Table 3
Features | Type 1 | Type 2 | Type 4 |
---|---|---|---|
Defect | Failure of α cells to secrete H+ and reclaim K+—non-functional H+/K+ antiporter | Failure to reabsorb HCO3− | Adrenal (deficiency of aldosterone) |
Collecting and distal renal tubules | Proximal renal tubules | Renal resistance to aldosterone | |
Can be associated with Fanconi syndrome | |||
Examples of Primary Causes | SLC4A1 gene; AE1 | Very rarely primary | WNK1 and WNK4 genes; inhibitors of NCC (Gordons) |
ATP6V0A4 and ATP6V1B1 genes; protein complex for vacuolar H+-ATPase (V-ATPase) | SLC4A4 gene: sodium bicarbonate cotransporter (NBCe1) | NR3C2 gene; mineralocorticoid receptor (PHA1) | |
SCNN1A, SCNN1B, SCNN1G genes; subunits of ENaC (PHA1) | |||
Corticosterone methyloxidase deficiency; PHA2 | |||
Secondary Causes | Sjogren’s, SLE, primary sclerosing cholangitis | Hypergammaglobulinaemia: multiple myeloma, amyloidosis | Aldosterone deficiency |
Sickle cell disease, chronic obstructive uropathy, hypogammaglobulinaemia, chronic liver disease, Wilson’s, post kidney transplant | Hereditary fructose intolerance, glycogen storage disease, cystinosis | Intrinsic tubular defect, e.g., interstitial fibrosis in diabetes associated chronic kidney disease or obstructive uropathy | |
Marfan’s, Ehlers-Danlos | Sjogren’s, SLE | Lupus nephritis causes tubulointerstitial damage and hyporeninaemic hypoaldosteronism | |
Drugs, e.g., lithium or ibuprofen, toluene abuse (glue sniffing) | Heavy metals, e.g., lead, cadmium | Low or resistant to aldosterone: adrenal insufficiency, amyloidosis, sickle cell disease, spironolactone, eplerenone, NSAIDs, ACEi, heparin, lovenox, amiloride, triamterene, trimethoprim, pentamidine, calcineurin inhibitors | |
Interstitial nephritis, vitamin D deficiency, secondary hyperparathyroidism, chronic hepatitis | Low renin: diabetic nephropathy, AIDS, intrinsic renal disease, IgM gammopathy, NSAIDs | ||
Drugs, e.g., tenofovir, ifosfamide, valproate, CA inhibitors, aminoglycosides, mercury | |||
Acidaemia | Yes—usually severe | Yes—less than type 1 | Mild when present |
Urine pH | >5.3 | <5.5 | <5.5 |
AE1, anion exchanger 1; NCC, thiazide sensitive sodium chloride cotransporter; ACEi, angiotensin converting enzyme inhibitors; AD, autosomal dominant; PHA1/2, pseudohypoaldosteronism type 1 and 2; ENaC, epithelial sodium channel; SLE, systemic lupus erythematosus; NSAIDs, non-steroidal anti-inflammatory drugs; AIDS, acquired immune deficiency syndrome; IgM, immunoglobulin M; CA, carbonic anhydrase.
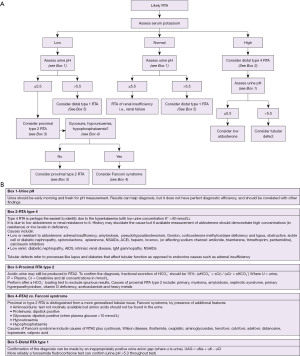
In RTA, one expects the presence of alkaline urine (pH >5.3) despite acidosis. However, this is not always the case. Early morning urine and fresh specimens are required, but distal (type 1) and hyperkalaemic (type 4) RTA can be associated with urine pH <5.5. Also, dehydration and urease containing bacteria in the urine can cause an apparent normal anion gap acidosis with alkaline urine mimicking RTA (14). Due to the possibility of spurious diagnoses in RTA, there are further tests to help confirm the diagnosis, such as: fractional excretion (FE) of bicarbonate, aldosterone concentration and urinary anion gap (Figure 5).
In these cases, a urinary anion gap can be useful to help distinguish different aetiologies:
Where u = urine, all concentrations in mmol/L, and all measurements can be performed on a random urine specimen. Normally, the gap is between 20–90 mmol/L (14). Ammonium (excretable acid) is excreted with chloride, therefore urinary chloride is an indirect measure of urinary ammonium. In distal (type 1) RTA, acid excretion is impaired, and when ammonium chloride is given (an acid load), the gap remains positive (whereas a normal response would be for the gap to drop and become negative) (14). There are risks with this test however, and gap calculation alone may be sufficient without using an acid load. High amounts of lithium, ketoacids and bicarbonate in the urine can interfere with this calculation.
Due to the danger and unpalatability of the ammonium chloride loading test, the furosemide fludrocortisone test is proposed as an alternative (25). This test is well tolerated and effectively provides a sodium load to the kidneys, with a simultaneous stimulation to reabsorb the sodium and hence excrete cations including protons. Failure to acidify the urine confirms distal RTA type 1 (16).
FE of bicarbonate is calculated by:
Where u = urine, s = serum, Cr = creatinine and all concentrations in mmol/L (sCr will need adjusting, divide by 1,000 if in µmol/L). In the presence of low serum bicarbonate, FE should be <5%. Repeating the calculation, using specimens taken under alkaline conditions, is preferred as this can prevent spurious values by providing a bicarbonate load to ensure the failure to reabsorb bicarbonate is manifested (FE >15% indicates type 2 RTA) (14). A bicarbonate loading test is performed by measuring hourly changes in serum HCO3− and creatinine and urinary pH, HCO3− and creatinine, whilst administering an intravenous infusion of sodium bicarbonate at a rate of 1 mmol/kg/h, until serum HCO3− approaches or reaches normal reference intervals (for example >24 mmol/L, see local ranges). At this point, a paired urinary pH >7.5 (or FE of HCO3− of >15%) is diagnostic of proximal RTA (patients without RTA, or with distal RTA, should demonstrate stable urinary pH). If fractional HCO3− excretion is <5%, proximal RTA can be excluded, but calculated values between 5–15% are unfortunately indeterminate.
Pregnancy and paediatrics
Pregnancy leads to haemodilution, changes in respiratory system, and reduction in bicarbonate concentration by 2–6 mmol/L (26) or 25% (27); effectively representing a fully compensated respiratory alkalosis. However, do not dismiss a low bicarbonate concentration automatically during pregnancy, particularly if they are presenting with possible metabolic acidosis, or breathlessness, as pregnant women lose some of their buffering capacity and are more sensitive to acidosis. In normal pregnancy, the pH may increase slightly by 0.01 at both the upper and lower end of the normal reference interval, also masking the onset of an acidosis (17).
Children approach adult concentration of carbon dioxide by 5 years of age, but neonates have a lower concentration of carbon dioxide, that increases over time, until they reach the age of 5 years (28). The pH and bicarbonate concentrations are assumed to be equivalent to adult normal ranges. Inborn errors of metabolism leading to acidosis (e.g., defects of organic acid, pyruvate of ketone body metabolism) are more likely to present in children than adults. Diagnostic schema for these conditions will not be presented here, but we would recommend using society guidelines or relevant articles (29).
Rare conditions
Carbon monoxide poisoning is a rare cause of mixed type A and B lactic acidosis. The normal range of carboxyhaemoglobin is between 1–3% (in smokers it can be up to 10%), and symptoms occur between 3–24% with death occurring at concentration >24% (30). If testing is not available for carboxyhaemoglobin, then diagnosis depends on history. Hydrogen cyanide (inhaled due to the burning of plastics, wools and silks) causes a type B lactic acidosis by binding cytochrome oxidase C (as does carbon monoxide) preventing the formation of ATP. If a patient presents with smoke inhalational injury, and oxygen therapy worsens the metabolic acidosis and hyperlactaemia, then one should consider cyanide poisoning (lactate levels correlate with the severity of cyanide poisoning) (31). Signs that indicate possible cyanide poisoning are:
- Signs consistent with poisoning, e.g., altered mental status, unconsciousness and convulsions;
- Evidence of smoke inhalation, e.g., soot in the mouth or expectoration;
- Metabolic acidosis on arterial blood sample with lactate >8 mmol/L (32).
Type B lactic acidosis occurs when various poisons affect the mitochondria, and lead to anaerobic respiration by a different mechanism. Causes of this include: ethanol combined with fasting, mitochondrial myopathies, biguanides, nucleoside reverse transcriptase inhibitors, poisons (methanol, ethylene glycol, salicylate, paracetamol, biguanides), and smoke inhalation. Propylene glycol is found in radiator fluid and is a very rare cause of lactic acidosis.
The larger the structure of a toxic alcohol, the less it will affect the anion and osmolar gap (e.g., a toxic dose of ethylene glycol may only change either by 5 mmol, whereas methanol would change it more significantly due to being smaller). Note also that alcohols may affect the osmolar gap more than the anion gap as they are non-ionic. However, the osmolar gap will reduce as the alcohol is metabolised, and the anion gap will increase as acids are formed by the metabolic process. Testing for toxic alcohols is not always available, and presentation depends on which type of alcohol has been consumed, and how much, if any, simultaneous ethanol. Ethanol will compete for metabolism by alcohol dehydrogenase, and can protect against the formation of toxic methanol and ethylene glycol metabolites including formic, oxalic, glycolic, and lactic acids. Concurrent ethanol ingestion can therefore maintain a raised osmolar gap for longer, as the other toxic alcohols remain unmetabolised (33).
If laboratory measurements are not available, then treatment of toxic alcohol consumption is recommended when one of the following criteria is met:
- Evidence or suspicion of 10 g of methanol or ethylene glycol (10 mL of 100%) and osmolar gap >10 mosmol/L;
- Suspected ingestion with either, an osmolar gap >10 mosmol/L or high anion gap metabolic acidosis with no other obvious cause (urinary oxalate crystals or lactate gap may help) (34,35).
Pyroglutamic acidosis is caused by 5-oxoproline (an endogenous organic acid) and results in a raised anion gap metabolic acidosis (this is detected in urine organic acid screens but not routinely or rapidly available). Causes of pyroglutamic acidosis include: inborn errors of metabolism, chronic paracetamol use, malnutrition, sepsis, antibiotics and renal impairment (10).
VIPomas, by causing high volume secretory diarrhoea, cause a hypokalaemic acidosis. This is because bicarbonate is secreted along with the sodium, potassium, and water (36). Urinary diversions are where the ileum or colon replace the urinary bladder. The urine causes the bowel to secrete sodium and bicarbonate, reabsorb ammonia, ammonium, hydrogen, and chloride, plus the ileum, in particular, reabsorbs potassium when used as a neobladder. The ammonium reabsorbed causes a hyperchloraemic metabolic acidosis. Using the colon as a bladder can lead to total body potassium depletion (37).
Conclusions
The investigation of acidosis can be challenging with many conditions affecting the homeostasis. However, a correctly interpreted arterial blood gas can give clear indication of where the problem may have originated. The diagnostic algorithms presented here will help the reader to systematically consider causes of acidosis. However, we appreciate that each patient case is different, and it may be appropriate to skip ahead to steps later in the algorithms, refer to other algorithms (e.g., alkalosis within this series), or even miss out steps entirely. These algorithms cannot replace specialist knowledge, experience, and local guidelines. Instead, they should act as diagnostic aids when assessing acidotic patients.
Acknowledgments
We would like to thank Professor Rousseau Gama for inspiring and inviting us to prepare this paper. All figures were created with BioRender.com.
Funding: None.
Footnote
Provenance and Peer Review: This article was commissioned by the editorial office, Journal of Laboratory and Precision Medicine for the series “Investigative Algorithms in Laboratory Medicine – Electrolytes and Acid/Base”. The article has undergone external peer review.
Reporting Checklist: The authors have completed the Narrative Review reporting checklist (available at https://jlpm.amegroups.com/article/view/10.21037/jlpm-22-9/rc).
Conflicts of Interest: All authors have completed the ICMJE uniform disclosure form (available at https://jlpm.amegroups.com/article/view/10.21037/jlpm-22-9/coif). The series “Investigative Algorithms in Laboratory Medicine – Electrolytes and Acid/Base” was commissioned by the editorial office without any funding or sponsorship. KS served as the unpaid Guest Editor of the series. ARS serves as Editor-in-Chief of Journal of Clinical and Experimental Dermatology. The authors have no other conflicts of interest to declare.
Ethical Statement: The authors are accountable for all aspects of the work in ensuring that questions related to the accuracy or integrity of any part of the work are appropriately investigated and resolved.
Open Access Statement: This is an Open Access article distributed in accordance with the Creative Commons Attribution-NonCommercial-NoDerivs 4.0 International License (CC BY-NC-ND 4.0), which permits the non-commercial replication and distribution of the article with the strict proviso that no changes or edits are made and the original work is properly cited (including links to both the formal publication through the relevant DOI and the license). See: https://creativecommons.org/licenses/by-nc-nd/4.0/.
References
- Adrogué HJ, Madias NE. Secondary responses to altered acid-base status: the rules of engagement. J Am Soc Nephrol 2010;21:920-3. [Crossref] [PubMed]
- Stewart PA. Modern quantitative acid-base chemistry. Can J Physiol Pharmacol 1983;61:1444-61. [Crossref] [PubMed]
- Hopkins E, Sanvictores T, Sharma S. Physiology, Acid Base Balance. [Updated 2021 Sep 14]. In: StatPearls. Treasure Island (FL): StatPearls Publishing; 2022 Jan.
- Martinu T, Menzies D, Dial S. Re-evaluation of acid-base prediction rules in patients with chronic respiratory acidosis. Can Respir J 2003;10:311-5. [Crossref] [PubMed]
- Dhondup T, Qian Q. Acid-Base and Electrolyte Disorders in Patients with and without Chronic Kidney Disease: An Update. Kidney Dis (Basel) 2017;3:136-48. [Crossref] [PubMed]
- Kraut JA, Madias NE. Serum anion gap: its uses and limitations in clinical medicine. Clin J Am Soc Nephrol 2007;2:162-74. [Crossref] [PubMed]
- Sasse SA, Berry RB, Nguyen TK, et al. Arterial blood gas changes during breath-holding from functional residual capacity. Chest 1996;110:958-64. [Crossref] [PubMed]
- O'Neill AV, Johnson DC. Transition from exercise to rest. Ventilatory and arterial blood gas responses. Chest 1991;99:1145-50. [Crossref] [PubMed]
- Herr RD, Swanson T. Pseudometabolic acidosis caused by underfill of Vacutainer tubes. Ann Emerg Med 1992;21:177-80. [Crossref] [PubMed]
- Davidson DF. Effects of contamination of blood specimens with liquid potassium-EDTA anticoagulant. Ann Clin Biochem 2002;39:273-80. [Crossref] [PubMed]
- Miller PM, Dufour DR. Extreme Hypernatremia with Markedly Increased Anion Gap. Clin Chem 2015;61:1422. [Crossref] [PubMed]
- Davis MD, Walsh BK, Sittig SE, et al. AARC clinical practice guideline: blood gas analysis and hemoximetry: 2013. Respir Care 2013;58:1694-703. [Crossref] [PubMed]
- Goodwin NM, Schreiber MT. Effects of anticoagulants on acid-base and blood gas estimations. Crit Care Med 1979;7:473-4. [Crossref] [PubMed]
- Byrne AL, Bennett M, Chatterji R, et al. Peripheral venous and arterial blood gas analysis in adults: are they comparable? A systematic review and meta-analysis. Respirology 2014;19:168-75. [Crossref] [PubMed]
- Walkey AJ, Farber HW, O'Donnell C, et al. The accuracy of the central venous blood gas for acid-base monitoring. J Intensive Care Med 2010;25:104-10. [Crossref] [PubMed]
- Habibzadeh F, Yadollahie M. Temperature correction of the calculated alveolar oxygen tension. Anesthesiology 1998;89:1042. [Crossref] [PubMed]
- Navaneethan SD, Mooney R, Sloand J. Pseudo-anion gap acidosis. NDT Plus 2008;1:94-6. [PubMed]
- Lolekha PH, Vanavanan S, Lolekha S. Update on value of the anion gap in clinical diagnosis and laboratory evaluation. Clin Chim Acta 2001;307:33-6. [Crossref] [PubMed]
- Gabow PA, Kaehny WD, Fennessey PV, et al. Diagnostic importance of an increased serum anion gap. N Engl J Med 1980;303:854-8. [Crossref] [PubMed]
- Kraut JA, Mullins ME. Toxic Alcohols. N Engl J Med 2018;378:270-80. [Crossref] [PubMed]
- Kreisberg RA. Lactate homeostasis and lactic acidosis. Ann Intern Med 1980;92:227-37. [Crossref] [PubMed]
- Palmer BF, Kelepouris E, Clegg DJ. Renal Tubular Acidosis and Management Strategies: A Narrative Review. Adv Ther 2021;38:949-68. [Crossref] [PubMed]
- Yaxley J, Pirrone C. Review of the Diagnostic Evaluation of Renal Tubular Acidosis. Ochsner J 2016;16:525-30. [PubMed]
- Haque SK, Ariceta G, Batlle D. Proximal renal tubular acidosis: a not so rare disorder of multiple etiologies. Nephrol Dial Transplant 2012;27:4273-87. [Crossref] [PubMed]
- Walsh SB, Shirley DG, Wrong OM, et al. Urinary acidification assessed by simultaneous furosemide and fludrocortisone treatment: an alternative to ammonium chloride. Kidney Int 2007;71:1310-6. [Crossref] [PubMed]
- Abbassi-Ghanavati M, Greer LG, Cunningham FG. Pregnancy and laboratory studies: a reference table for clinicians. Obstet Gynecol 2009;114:1326-31. [Crossref] [PubMed]
- Teasdale S, Morton A. Changes in biochemical tests in pregnancy and their clinical significance. Obstet Med 2018;11:160-70. [Crossref] [PubMed]
- Adeli K, Higgins V, Trajcevski K, et al. The Canadian laboratory initiative on pediatric reference intervals: A CALIPER white paper. Crit Rev Clin Lab Sci 2017;54:358-413. [Crossref] [PubMed]
- Schillaci LP, DeBrosse SD, McCandless SE. Inborn Errors of Metabolism with Acidosis: Organic Acidemias and Defects of Pyruvate and Ketone Body Metabolism. Pediatr Clin North Am 2018;65:209-30. [Crossref] [PubMed]
- Hampson NB, Hauff NM. Carboxyhemoglobin levels in carbon monoxide poisoning: do they correlate with the clinical picture? Am J Emerg Med 2008;26:665-9. [Crossref] [PubMed]
- Baud FJ. Cyanide: critical issues in diagnosis and treatment. Hum Exp Toxicol 2007;26:191-201. [Crossref] [PubMed]
- Lawson-Smith P, Jansen EC, Hyldegaard O. Cyanide intoxication as part of smoke inhalation--a review on diagnosis and treatment from the emergency perspective. Scand J Trauma Resusc Emerg Med 2011;19:14. [Crossref] [PubMed]
- Kurtz I. Renal Tubular Acidosis: H+/Base and Ammonia Transport Abnormalities and Clinical Syndromes. Adv Chronic Kidney Dis 2018;25:334-50. [Crossref] [PubMed]
- Musa D. Trust Guideline for the Management of Toxic Alcohols (Ethylene glycol and methanol) with Fomepizole [Internet]. 2021. Available online: https://www.nnuh.nhs.uk/publication/toxic-alcohols-ethylene-glycol-and-methanol-with-fomepizole-management-of-ca5141-v2/ (accessed 23 December 2021).
- Toxbase. Ethylene Glycol [Internet]. Available online: https://www.toxbase.org/?ReturnUrl=%2FPoisons-Index-A-Z%2FE-Products%2FEthylene-Glycol%2F (accessed 23 December 2021).
- Schwab M. editor. Verner-Morrison Syndrome. In: Encyclopedia of Cancer. Berlin, Heidelberg: Springer Berlin Heidelberg; 2009:3165.
- Mundy AR. Metabolic complications of urinary diversion. Lancet 1999;353:1813-4. [Crossref] [PubMed]
Cite this article as: Allen GT, Flowers KC, Shipman AR, Darragh-Hickey C, Kaur S, Shipman KE. Investigative algorithms for disorders affecting acidosis: a narrative review. J Lab Precis Med 2022;7:24.