Investigative algorithms for disorders affecting plasma potassium: a narrative review
Introduction
Potassium is a major intracellular ion and is one of the most essential nutrients required for the functioning of normal physiology. The constant activity of sodium-potassium adenosine triphosphate (ATP) pumps within almost every cell membrane maintains a predominantly intracellular potassium concentration of 100 to 150 mmol/L (1 mmol/L is equal to 1 mEq/L) (1), with an extracellular concentration of approximately 3.5 to 5.3 mmol/L (2). This potassium concentration difference between the intracellular and extracellular compartments contributes to an electrochemical potential across cell membranes, and this can be manipulated to produce charges for neuronal signalling and muscular contractions.
Potassium homeostasis is maintained by balancing potassium intake in the diet with excretion, and via altering the distribution around the body (Figure 1) (3). The average human consumes approximately 80 mmol of potassium per day (4). Ninety percent of ingested potassium is absorbed from the diet, and the remaining 10% is excreted in the faeces (5). Of the absorbed potassium, almost all of it is excreted renally (Figure 2) (5). Changes in physiological states including some presentations of acid base disturbances, and the presence of hormones including insulin and adrenaline, can lead to potassium shifting between the extracellular and intracellular compartments. As only approximately 2% of the total body stores of potassium are in the extracellular compartment (3,5), minor disturbances in renal excretion, or maintenance of intracellular potassium concentration, can lead to profound and significant changes in the extracellular concentration. If severe enough, this can be a medical emergency due to the resulting risk of cardiac arrhythmias and cardiac arrest (2).
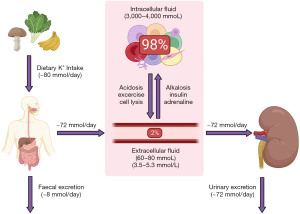
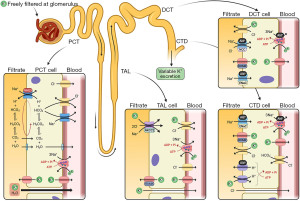
Plasma potassium analysis can be a useful tool in assessing patients who are at risk of hyperkalaemia or hypokalaemia. However, potassium analysis is prone to providing results that are not an accurate reflection of the true in vivo status, and being aware of these is imperative for appropriate patient management. Although many excellent guidelines for investigating potassium disturbances have been previously published, many are very long and/or require significant clinical assessment. The following algorithms primarily aim to provide a rough guide on using laboratory diagnostics to evaluate the causes of potassium disturbances from the laboratory perspective. This will hopefully allow laboratory personnel to add diagnostic value to individual cases, even if lacking some of the clinical information. However, clinicians may still find these algorithms useful when organising their thoughts on how to investigate patients with a potassium disturbance. We present the following article in accordance with the Narrative Review reporting checklist (available at https://jlpm.amegroups.com/article/view/10.21037/jlpm-22-10/rc).
Methods
The narrative literature review was created by searching Medline, Google Scholar, OMIM and seminal texts. The diagnostic algorithms were then created using the information gathered for the literature review. The literature was searched over the period September 2021 to January 2022. The language was restricted to English. For further information please see Table 1.
Table 1
Items | Specification |
---|---|
Date of search (specified to date, month and year) | September 2021 to January 2022 |
Databases and other sources searched | Medline, Google Scholar, OMIM |
Search terms used (including MeSH and free text search terms and filters). Examples include: | Potassium, Hyperkalaemia, Hypokalaemia, Drugs, Investigations, Algorithms, Guidelines, Diagnosis, Causes, Aetiology, Human |
Timeframe | From database inception to January 2022 |
Inclusion and exclusion criteria (study type, language restrictions, etc.) | All papers and reviews were included restricted to English |
Selection process (who conducted the selection, whether it was conducted independently, how consensus was obtained, etc.) | Kade C. Flowers and Kate E. Shipman conducted initial search, with refinement by all other authors to obtain consensus and agreement |
Any additional considerations, if applicable | Seminal texts were also searched and the references of important articles and texts were obtained and checked for relevance |
Potassium homeostasis
An in-depth review of potassium homeostasis is beyond the scope of this article and is available elsewhere (2-7). However, in brief, potassium is freely filtered at the glomerulus and 90% is reabsorbed back into the blood in the proximal convoluted tubule (PCT) via paracellular passive diffusion and solvent drag. Potassium reabsorption in the thick ascending limb (TAL) occurs via transcellular and paracellular mechanisms. Cells in the TAL contain sodium potassium chloride co-transporters (NKCC2), which are the transporters that loop diuretics block to increase water excretion. Potassium secretion begins in the early convoluted tubule and distal convoluted tubule (DCT) via renal outer medullary potassium channels (ROMK). In states of potassium depletion, potassium reabsorption is increased by upregulating ATP dependent proton-potassium transporters in the collecting duct (CTD). Figure 2 provides a summary of renal potassium handling (2).
The rate of potassium secretion in the distal nephron varies depending on physiological requirements. In the DCT and CTD, the hormone aldosterone acts on the sodium-chloride cotransporter (NCC), epithelial sodium (ENaC) and proton uniporter channels on the apical (filtrate) membrane—and sodium-potassium pumps on the basolateral (blood) membrane—to increase sodium (and therefore water) reabsorption from the filtrate (Figure 2) (8). Refer to the sodium article within this series for more information on the physiology of aldosterone secretion. Increased sodium reabsorption from aldosterone action increases the electronegativity of the filtrate, favouring potassium excretion. Aldosterone also has a direct effect on the apical membrane to increase potassium permeability, leading to more potassium excretion into the filtrate (7).
Hyperkalaemia
Hyperkalaemia is a common electrolyte disturbance and can cause life threatening dysrhythmias and cardiac arrest (9). The population wide prevalence of hyperkalaemia is unknown, but it is present in approximately 4% of elderly patients aged >65 years old who are admitted to hospital (10), and as many as 54% of chronic kidney disease (CKD) patients (11). There are many recognised clinical conditions, and spurious causes, that can cause hyperkalaemia. Here, we present a basic algorithm that demonstrates a laboratory diagnostics approach to patients presenting with hyperkalaemia (Figure 3).
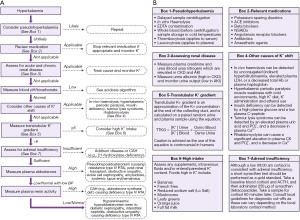
Pseudohyperkalaemia
Pseudohyperkalaemia is a high potassium result that does not reflect the true in vivo potassium status, so can be considered spurious for practical and clinical purposes. Pseudohyperkalaemia should always be considered in every case of hyperkalaemia, especially if the patient is asymptomatic, not on any relevant drugs, typical electrocardiogram (ECG) presentations (Figure 4) are absent, and the estimated glomerular filtration rate (eGFR) is >90 mL/min/1.73 m2 (12). Failure to correctly identify pseudohyperkalaemia can lead to inappropriate treatment, or delayed treatment of cases of true hypokalaemia. An algorithm is presented for investigating pseudohyperkalaemia if this is suspected (Figure 5).
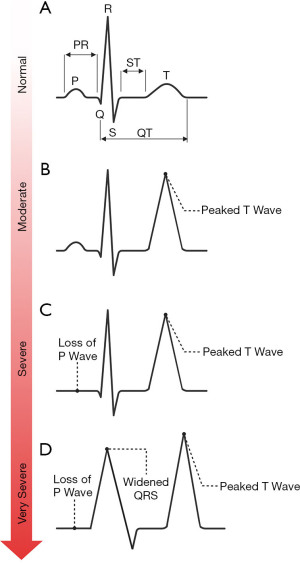
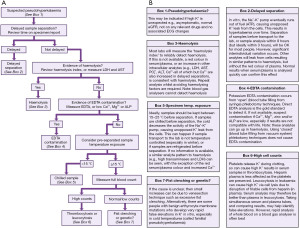
As whole blood clots when collecting serum samples, potassium is released from platelets into the serum. Importantly, this means that potassium concentrations in serum samples are approximately 0.4 mmol/L higher than they are in plasma and whole blood samples (13). Serum samples should therefore have a higher reference range than plasma and whole blood samples (14). Pseudohyperkalaemia has been defined as a serum potassium concentration that is >0.4 mmol/L than the plasma potassium in a sample that is collected at the same time and analysed within one hour (15). However, this incorrectly suggests that pseudohyperkalaemia is exclusive to serum samples and does not occur in plasma samples.
If pseudohyperkalaemia is suspected, a repeat sample may confirm the diagnosis. It may be appropriate to send a different sample type for the repeat or measure the potassium on whole blood using a blood gas analyser, depending on what the suspected contributing factor is. However, caution is advised as blood gas analysers cannot detect the presence of in vitro haemolysis (16). If repeat and/or alternative sample analysis for potassium still provides a high result, the result is much more likely to be real and reflect the true in vivo potassium status (including a true hyperkalaemia caused by in vivo haemolysis).
Medication causes of hyperkalaemia
After exclusion of possible pseudohyperkalaemia, it is essential to determine the urgency of the clinical situation. If ECG changes associated with hyperkalaemia (Figure 4) (17) are present (which in practice should be determined before consideration of pseudohyperkalaemia), it is likely that urgent treatment is required (follow local guidelines) in order to stabilise the patient before a more thorough investigation of the cause can commence.
A quick and important subsequent investigation should include an assessment of the drug history. However, this relies on access to the patient’s medical notes or the effective use of clinical details on the request. A variety of drugs (Figure 3) can directly cause hyperkalaemia or exacerbate mild hyperkalaemia from other causes, via multiple mechanisms (18). If a patient has hyperkalaemia and is taking a drug that causes hyperkalaemia, it may be appropriate to stop the medication or reduce its dose and monitor the blood potassium in response (18).
Acute and chronic renal disease
Good renal function is essential for matching potassium excretion with potassium intake (Figure 1). If acute or chronic renal disease is present, potassium frequently accumulates in the extracellular compartment and leads to hyperkalaemia. If acute or chronic renal disease is suspected, the quickest and simplest method to assess renal function is to measure the serum/plasma creatinine concentration and monitor the urine output. An increase in creatinine of 26 µmol/L (0.3 mg/dL) within 48 hours, and/or an increase in creatinine >1.5 times a baseline creatinine result from the previous 7 days, and/or <0.5 mL/kg/h urine output for six hours, is diagnostic of an acute kidney injury (AKI) (19). Creatinine is also useful for calculating the eGFR for the assessment of CKD. CKD can be defined by any of the following: eGFR <60 mL/min/1.72 m2, 24-hour albuminuria ≥30 mg/24 h, albumin to creatinine ratio (ACR) ≥3 mg/mmol (≥30 mg/g), urine sediment abnormalities, imaging and histology abnormalities, electrolyte disturbances due to a tubular disorder and/or history of kidney transplantation (20). Urea and cystatin C are other markers of renal function, but they have their own limitations or are not well established in routine practice (21). Once an AKI or CKD has been diagnosed, potential causes need to be investigated (e.g., dehydration, organ failure, drugs, diabetes, hypotension, trauma, autoimmunity).
Acidosis
Some presentations of acidosis can cause hyperkalaemia due to intracellular and extracellular redistribution. Blood gas analysis, and/or laboratory plasma bicarbonate analysis, can aid in the identification of acidosis (see article in this series on acidosis).
Other causes of potassium shift
Unlike in vitro haemolysis, the potassium result generated after in vivo haemolysis can be a true reflection of the in vivo potassium status. Conditions that can cause in vivo haemolysis include haemolytic anaemia, transfusion reactions, haemoglobinopathies, thalassaemia, hereditary spherocytosis, gluose-6-phosphate (G6P) and pyruvate kinase deficiencies and disseminated intravascular coagulation (22). The physical in vivo destruction of red blood cells (RBCs) can occur in patients with mechanical heart valves (23). As free haemoglobin is released during in vivo haemolysis, it can be detected by the laboratory using the same spectrophotometric methods that detect in vitro haemolysis. Other RBC contents, including potassium and lactate dehydrogenase (LDH), are also released into the plasma during in vivo haemolysis as they are during in vitro haemolysis (24). In vivo haemolysis can therefore be difficult to biochemically distinguish from in vitro haemolysis, the former of which requires treatment and the latter may not be clinically relevant.
However, an increase in blood unconjugated (indirect) bilirubin is consistent with, but not specific to, in vivo haemolysis. Additionally, total haemoglobin, and serum/plasma total haptoglobin, are decreased in in vivo haemolysis, but remain the same compared to the RBC count and haematocrit in in vitro haemolysis (25). This is because during in vivo haemolysis, haptoglobin binds to free haemoglobin and the complexes are quickly excreted, but this excretion cannot occur after a sample is taken (24). However, haptoglobin is a positive acute phase reactant, so caution is advised when interpreting results during inflammation (26).
Rhabdomyolysis is a rapid dissolution of damaged skeletal muscle that causes intracellular release of potassium. Rhabdomyolysis is most commonly caused by physical muscle injury but can also occur after strenuous exercise and drug toxicity. Rhabdomyolysis can cause an AKI, which can in turn exacerbate a hyperkalaemia (27). Increased myoglobin in the urine, a high plasma creatine kinase (CK) and phosphate, and a low plasma calcium, are frequently observed in patients with rhabdomyolysis. Although urine myoglobin is rarely measured in practice, a brown urine colour, and/or a positive urine dipstick for blood without RBC sediment, is suspicious for myoglobinuria (28).
Tumour lysis syndrome (TLS) is caused by a rapid destruction of tumour cells (typically from blood malignancies) after treatment. This results in a release of potassium, phosphate and uric acid into the plasma with an increased risk of kidney injury, and a decreased calcium (29).
Insulin is a powerful stimulant of cellular uptake of potassium. Therefore, conditions which cause a deficiency of insulin (e.g., diabetes, pancreatic trauma) can cause hyperkalaemia as well as more obvious hyperglycaemia. Plasma glucose analysis can help to identify an initial disturbance in glucose metabolism. C-peptide is a part of the precursor to insulin called proinsulin, and is secreted into the plasma in equimolar amounts to insulin (30). Plasma or urine C-peptide analysis can help to determine if there is any endogenous insulin secretion, which can help to differentiate between insulin deficiency or resistance. C-peptide analysis is usually more appropriate than direct insulin analysis as it has a longer half-life for detection, and is not metabolised as extensively by the liver (30). If not available, those with risk factors for diabetes mellitus type 2, features of metabolic syndrome, or a history of pancreatic injury with proven hyperglycaemia, have a high chance of having insulin deficiency.
Assessing renal response to hyperkalaemia
The analysis of urine potassium concentration is of limited value in the assessment of hyperkalaemia, as urinary excretion is dependent on intake. Additionally, methods to assess if the kidneys are responding appropriately to hyperkalaemia, including 24-hour urine collections for potassium excretion, have specific limitations (31). As a result of the kidney’s ability to greatly adapt excretion, excessive intake of potassium is a rare cause of hyperkalaemia in the absence of AKI and CKD (which should be ruled out before considering urine potassium measurement). For information purposes however, a random urine potassium concentration of <20 mmol/L during hyperkalaemia is inappropriately low, which may be useful to confirm impaired renal excretion (e.g., from AKI or CKD) as a cause.
The transtubular potassium gradient (TTKG) is a calculation that estimates the concentration of potassium in the filtrate of the collecting duct in relation to the blood (32). As the TTKG equation was validated in rats, and it is only valid when the urine osmolality is greater than the plasma osmolality (which is frequently not the case during AKI), caution is advised when using TTKG in humans (32). It is perhaps more appropriate to assess the potassium intake, and then if not relevant, go straight for the assessment of adrenal function and/or aldosterone response.
However, a TTKG ≥6 suggests that the kidneys are responding appropriately to secreted aldosterone in the presence of hyperkalaemia. Therefore, excess potassium intake via the diet (enteral or parenteral) or IV fluids could be an explanation (32). A TTKG <6 suggests that the kidneys are not appropriately secreting potassium in the presence of hyperkalaemia (32), meaning that there may be a problem with the bioactivity of aldosterone or its secretion. In cases where direct mineralocorticoid measurement is problematic or inconclusive, the TTKG also has utility in differentiating mineralocorticoid deficiency from mineralocorticoid resistance by assessing its change after administration of exogenous mineralocorticoid (32).
Adrenal insufficiency
The action of aldosterone is to increase sodium reabsorption from the renal filtrate, at the expense of increased potassium and proton excretion in order to maintain electroneutrality. As aldosterone is produced in the adrenal glands, a primary dysfunction of the adrenal gland can result in the insufficient secretion of aldosterone, decreasing the renal excretion of potassium which can cause hyperkalaemia. Hyperkalaemia rarely results from secondary adrenal insufficiency [impaired adrenocorticotropic hormone (ACTH) secretion from the pituitary] as aldosterone secretion is primarily regulated by the renin-angiotensin-aldosterone system (RAAS)—which remains intact in these cases—and hyperkalaemia is in itself a potent direct stimulator of aldosterone secretion (33).
Adrenal function can be assessed by the ability to secrete cortisol. Cortisol exhibits a diurnal variation, where it is highest in the morning and lowest in the evening (34). A blood cortisol concentration less than a defined cut-off (check local guidelines) on a sample taken at around 09:00 may be inappropriately low, suggesting that the adrenal gland is not functioning correctly. This should be confirmed by a gold standard short synacthen (synthetic ACTH) test (SST), which directly stimulates the adrenal gland to produce cortisol to assess if the response is adequate (Figure 3) (35). As cortisol cut-offs are dependent on the cortisol method a laboratory uses and the local population, it is important to always check local guidelines to avoid misdiagnosis.
There are important caveats to consider when interpreting SST results. For example, long term secondary adrenal insufficiency, and prolonged exogenous steroid replacement/abuse, can give SST results similar to primary adrenal insufficiency due to adrenal atrophy. Use of oestrogen (e.g., contraceptive pill or hormone replacement therapy) will increase the cortisol binding globulin concentration (35). This will therefore increase total cortisol, giving spuriously normal cortisol measurements in deficiency states (hormones need stopping six weeks prior to cortisol analysis). Primary adrenal insufficiency can be a medical emergency, therefore the risk of false negatives is concerning. See the sodium article within this series for more information on interpreting SSTs. A sufficient cortisol response after an SST does not rule out aldosterone deficiency (primary hypoaldosteronism) or RAAS abnormalities, where aldosterone and renin activity measurement may be indicated.
Aldosterone and renin activity measurement
Aldosterone
An elevated extracellular potassium level directly stimulates the adrenal glands to secrete aldosterone (33). Aldosterone should therefore be appropriately elevated during hyperkalaemia in an attempt to increase urinary excretion. If aldosterone is appropriately elevated but reduced urinary potassium excretion has been demonstrated or is suspected, it is possible that there is defective aldosterone action as a consequence of pseudohypoaldosteronism (PHA) (further information in the rare section), sickle cell nephropathy, post renal transplant, obstructive uropathy, amyloidosis or systemic lupus erythematosus (1).
Renin activity
If aldosterone is inappropriately low during hyperkalaemia, and hypotension is present, renin activity should be elevated if the kidneys are able to appropriately secrete renin. Appropriate hyperreninaemia may mean that there is an aldosterone synthesis defect (primary hypoaldosteronism), which can occur in patients with congenital adrenal hyperplasia (CAH) (e.g., 21-hydroxylase, 3β-hydroxysteroid dehydrogenase and aldosterone synthase deficiencies, although 21-hydroxylase and 3β-hydroxysteroid dehydrogenase deficiencies are unlikely if an SST demonstrates good cortisol reserve). Steroid synthesis in the adrenal glands can be affected by multiple CAH causing mutations in enzymes (Figure 6) (36). It is important to note that 11-deoxycorticosterone possesses weak mineralocorticoid activity, so enzyme deficiencies that significantly increase its level (e.g., 11β-hydroxylase and 17α-hydroxylase) can conversely cause hypertension and hypokalaemia (37). The diagnosis of CAH can vary depending on the enzyme defect. El-Maouche et al. (38) have published a detailed review of CAH if more information is required. If plasma renin activity is also inappropriately low, this is termed hyporeninaemic hypoaldosteronism. Hyporeninaemic hypoaldosteronism due to non-medication causes during hyperkalaemia is usually acquired from diabetic nephropathy, interstitial nephritis or obstructive uropathy (1).
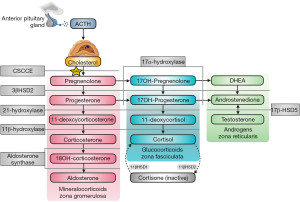
Interpreting aldosterone and renin activity results
The interpretation of aldosterone and renin activity can be difficult as the biochemical presentations can sometimes overlap. Additionally, antihypertensive drugs and patient posture will also affect the results, and freezing the sample before analysis of the unstable renin activity can be problematic (39). Potassium sparing and potassium-wasting diuretics should be stopped four weeks prior to the test (39). β-adrenergic blockers, α-2 agonists (e.g., clonidine, α-methyldopa), non-steroidal anti-inflammatory drugs, angiotensin-converting enzyme inhibitors, angiotensin receptor blockers, renin inhibitors and dihydropyridine calcium channel antagonist should be stopped at least two weeks before the test (39). It is worth noting that the aldosterone to renin ratio (ARR) can be less affected by these preanalytical factors than the isolated measurements.
Rare causes of hyperkalaemia
Hyperkalaemic periodic paralysis (HPP) is a rare autosomal dominant disorder of the sodium channel in skeletal muscle. This can cause muscle weakness or paralysis after exposure to precipitating factors including cold environments, fasting, alcohol consumption and potassium ingestion. This can result in hyperkalaemia via intracellular (muscle) release (40).
PHA is a rare cause of hyponatraemic hyperkalaemic acidosis due to resistance to aldosterone’s effects (mimicking adrenal insufficiency). Type I PHA has autosomal dominant (mineralocorticoid receptor mutation) or recessive (epithelial sodium channel subunit mutations) inheritance sub types (41). Type II PHA, also known as Gordon syndrome, presents as a mild hyperchloraemic metabolic acidosis that responds to thiazides. Type II PHA is typically autosomal dominant, with mutations in the WNK family of serine-threonine kinases that regulate other channels, e.g., NCC, ROMK, ENaC, and Cl transporters (42).
Hypokalaemia
Hypokalaemia is a common electrolyte disturbance and can lead to significant symptoms including fatal arrythmias. Mild hypokalaemia (3.0–3.4 mmol/L) can be found in almost 14% of outpatients, 20% of inpatients and up to 80% of patients who take diuretics (43). Chronic presentations of hypokalaemia can be a diagnostic challenge, but causes can be crudely split up into reduced intake, cellular shift and excessive excretion. Here, we present an algorithm focussing on the laboratory investigation of patients presenting with hypokalaemia (Figure 7).
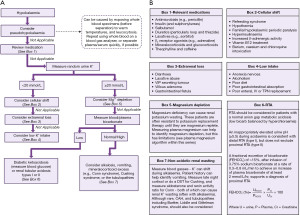
Pseudohypokalaemia
Pseudohypokalaemia is a low potassium result that does not reflect the true in vivo potassium status. Similarly to pseudohyperkalaemia, pseudohypokalaemia can be considered ‘fake’ for practical and clinical purposes. Although pseudohypokalaemia is rarer than pseudohyperkalaemia, it still poses a significant risk if not identified. Pseudohypokalaemia should be considered if the patient is asymptomatic, they are not on any relevant medications, and associated ECG changes (Figure 8) are absent.
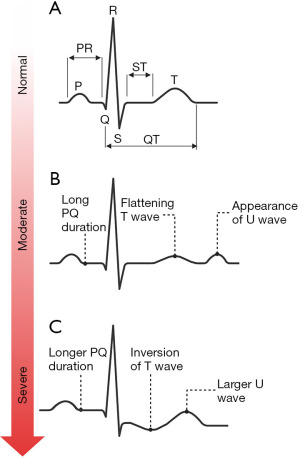
There are two main causes of pseudohypokalaemia. In direct contrast to cold temperatures that can cause pseudohyperkalaemia, warm temperatures (particularly in the summer) can increase the activity of sodium-potassium pumps (44). This can lead to increased movement of extracellular potassium to the intracellular compartment after the sample has been taken, thus decreasing the serum/plasma potassium concentration. This can occur until the glucose content in the sample has been expended, at which point ATP production will decrease and pseudohyperkalaemia will ensue. The solution to this is to not expose precentrifuged samples to temperatures >25 ℃, temperature control samples during transport, or centrifuge samples prior to laboratory transportation.
The second factor that can cause pseudohypokalaemia is leucocytosis (common in leukaemia), which can, confusingly, also cause pseudohyperkalaemia as previously described in Figure 5B. The metabolism of leucocytes can continue in vitro, leading to excess potassium being pumped intracellularly when there is an excess of white blood cells (WBCs) (45). Plasma samples are often more affected by warm temperatures and leucocytosis than serum samples, as there is more surface area contact of plasma with free blood cells than there is in serum with clotted cells. If pseudohypokalaemia from leucocytosis is suspected, rapid analysis of whole blood on a blood gas analyser is best to obtain the most accurate result. However, if this is not possible, rapid separation of plasma/serum from the cells can help to negate this effect.
Medication causes of hypokalaemia
After exclusion of possible pseudohypokalaemia, it is essential to determine the urgency of the clinical situation. If ECG changes associated with hypokalaemia (Figure 8) (17) are present (which in practice should be determined before consideration of pseudohypokalaemia), it is likely that urgent treatment is required (follow local guidelines) in order to stabilise the patient before a more thorough investigation of the cause can commence.
Considering relevant medications that may be contributing to hypokalaemia is the next thing that can be quickly done to help identify a cause (if there is access to this information). If a particular medication is a likely cause of the hypokalaemia, it may be appropriate to stop it or reduce the dose, and monitor the response by measuring blood potassium periodically until normal (46).
Urine potassium analysis
If there are no obvious medication causes of hypokalaemia, a quick and useful next step is to measure the urine potassium on a random urine sample. If the random urine potassium is <20 mmol/L, this suggests that the kidneys are appropriately reabsorbing potassium from the renal filtrate. If the random urine potassium concentration is ≥20 mmol/L, this suggests that the kidneys are failing to reabsorb potassium from the renal filtrate leading to inappropriate loss. A potassium to creatinine ratio of >1.5 mmol/mmol, or a 24-hour urine collection demonstrating renal potassium excretion of >15 mmol/24 h, can be useful alternatives to a random urine potassium sample to show inappropriate excretion (43). However, 24-hour urine collections can be cumbersome for patients.
Random urine potassium <20 mmol/L (appropriate renal reabsorption)
If the kidneys are appropriately reabsorbing potassium during hypokalaemia (random urine potassium of <20 mmol/L), the cause is more likely to be cellular shift, extrarenal loss or inadequate intake.
Cellular shift (compartment redistribution)
Many factors can cause potassium to shift from the extracellular compartment to the intracellular compartment. Refeeding syndrome is a potentially fatal complication when chronically malnourished people start to receive nutrition. During prolonged periods of malnourishment, the intracellular content of potassium, magnesium, phosphate and thiamine (vitamin B1) can become depleted, but blood concentrations can be normal. Upon refeeding, hormonal responses (including insulin) can cause a rapid shift of these analytes into cells due to a switch from catabolism to anabolism, causing dangerous reductions in their extracellular concentrations (47).
Conditions which can cause hyperinsulinism independent of plasma glucose concentration (e.g., insulinoma of the pancreas) can cause hypokalaemia due to stimulation of sodium-potassium pumps by insulin. Hyperinsulinism can be demonstrated by an inappropriately detectable insulin and c-peptide blood concentration during hypoglycaemia.
Increased endogenous β2 adrenergic activity can also increase the activity of sodium-potassium pumps. This occurs in excess secretion of catecholamines (e.g., adrenaline and noradrenaline) that happens in conditions such as phaeochromocytoma and paraganglioma (48). These conditions are usually accompanied by hypertension that is resistant to drug therapy and can be detected biochemically by elevated metadrenalines in plasma or 24-hour urine samples (48). Plasma metadrenalines have slightly better sensitivity than 24-hour urine metadrenalines, but both perform well enough for clinical use (49). However, plasma metadrenalines require strict collection conditions that can usually only be achieved in inpatient or outpatient settings and are more prone to false positive results than 24-hour urine metadrenalines.
Vitamin B12 replacement as a treatment for deficiency can cause hypokalaemia due to the increased intracellular requirement for haematopoiesis (50). Toxicity from barium, caesium and chloroquine are also associated with hypokalaemia via compartment redistribution.
Extrarenal potassium loss
Hypokalaemia can also result from excess potassium loss via the gastrointestinal (GI) tract. Perhaps the most common condition that can contribute to this is diarrhoea, and guidelines exist for its investigation (51). Although much more rare, vasoactive intestinal peptide secreting tumours (a neuroendocrine tumour), villous adenoma and GI fistulas should also be considered as causes of excess GI tract potassium loss.
Low potassium intake
Hypokalaemia due to poor intake is less common than hypokalaemia from redistribution and loss. Despite this, low intake should be particularly considered in patients who are at risk of poor nutritional intake, including the elderly, malnourished, alcoholics and drug addicts.
Random urine potassium ≥20 mmol/L (inappropriate renal loss)
If the kidneys are failing to reabsorb potassium from the filtrate (random urine potassium of ≥20 mmol/L during hypokalaemia), the cause is more likely to be from magnesium depletion, a tubulopathy, renal tubular acidosis (RTA) type I or II, diabetic ketoacidosis (DKA), vomiting, alkalosis, or mineralocorticoid or glucocorticoid excess.
Magnesium deficiency
Magnesium deficiency is an important cause and precipitating factor in approximately 40% of clinically significant hypokalaemia cases (52). Intracellular magnesium within cells of the distal nephron is important for inhibiting apical (filtrate) membrane ROMK channels, which usually prevents excessive potassium excretion into the renal filtrate. Magnesium deficiency leads to less intracellular magnesium and less inhibition of ROMK channels, resulting in increased urinary potassium excretion (53,54). It is important to identify magnesium deficiency as concurrent hypokalaemia can be refractory to treatment until the magnesium deficiency has been addressed (53). However, magnesium deficiency can be difficult to identify from the blood concentration alone, as this may not be representative of tissue stores (see magnesium article in this series of linked articles).
Acidosis
Acidosis can usually be detected by a low serum bicarbonate concentration, or a decreased pH and calculated bicarbonate via blood gas analysis (see acidosis article in this series of linked articles). During acidosis, hyperkalaemia is more common than hypokalaemia due to the shift of potassium from the intracellular compartment to the extracellular compartment. However, RTA types I and II, and DKA, can cause hypokalaemia due to increased renal excretion of potassium. The measurement of blood glucose and ketones can quickly help to identify if DKA is causing total body potassium depletion, and hypokalaemia, via osmotic diuresis (55). However, hypokalaemia is often not present during DKA due to hypoinsulinaemic potassium redistribution, and if present, it is usually not severe (55). Hypokalaemia during DKA can pose a significant clinical risk upon treatment with insulin.
RTA type I or II should be considered in patients with a normal anion gap metabolic acidosis (low bicarbonate balanced by hyperchloraemia—see acidosis and chloride articles in linked series).
Non-acidotic renal potassium wasting
Alkalosis can be identified by blood gas analysis or a high serum bicarbonate, and can cause hypokalaemia due to compartment redistribution and renal excretion (see alkalosis article in linked series). Vomiting can cause hypokalaemia by increasing urinary potassium excretion due to the increased load of filtered bicarbonate and hypovolaemia induced hyperaldosteronism (56). Mineralocorticoid excess, and the renal tubulopathies (e.g., Bartter, Liddle, and Gitelman syndromes), can cause hypokalaemia and should be considered if more common causes have been excluded. Mineralocorticoid excess can occur in Conn syndrome, CAH and apparent mineralocorticoid excess (AME). Conn syndrome (primary hyperaldosteronism) leads to autonomous adrenal aldosterone secretion. Up to 90% of patients with Conn syndrome are normokalaemic, but an ARR should still be calculated as a screen if it is suspected (particularly if the patient is hypertensive) (57). ARR cut-offs can vary depending on the units used and local laboratory methods (check local guidelines). A high ARR primarily suggests that the renin concentration is low (57), and may be consistent with Conn syndrome. It may be appropriate to do further testing such as a saline infusion test or fludrocortisone suppression test, both of which aim to appropriately suppress circulating aldosterone. Failure to suppress aldosterone during these tests is consistent with Conn syndrome (but caution is advised as multiple factors can complicate interpretation) (57).
Excess mineralocorticoid receptor stimulation via other mechanisms can also cause hypokalaemia. As 11-deoxycorticosterone (Figure 6) has weak mineralocorticoid activity, 11β-hydroxylase and 17α-hydroxylase deficiencies can cause hypokalaemia as these deficiencies can significantly increase its concentration (38). 11β-hydroxylase and 17α-hydroxylase deficiencies can cause hyporeninaemic hypoaldosteronism, as renin is suppressed by hypertension and aldosterone synthesis is reduced (38). Although cortisol has less affinity for mineralocorticoid receptors than glucocorticoid receptors, Cushing syndrome can result in high enough cortisol concentrations to significantly stimulate mineralocorticoid receptors. Cushing syndrome can be diagnosed by a dexamethasone suppression test, where failure to suppress the 09:00 am cortisol to <50 nmol/L, after the administration of 1 mg of dexamethasone the night before, makes a diagnosis possible (58). Late night salivary cortisol and 24-hour urine cortisol are also useful tests to diagnose Cushing syndrome. It is recommended that local guidelines are consulted if Cushing syndrome is suspected.
Bartter, Liddle and Gitelman syndromes are inherited conditions causing dysfunction in renal reabsorption and secretion of electrolytes. Hypokalaemia can be a manifestation of all three of these tubulopathies (see alkalosis article in this series of linked articles).
Rare causes of hypokalaemia
Hypokalaemic periodic paralysis is a rare hereditary condition that can cause sudden hypokalaemia, muscle weakness and paralysis due to defective calcium or sodium channels in skeletal muscle cells (59).
Although very rare, a deficiency of 11β hydroxysteroid dehydrogenase type II (11β-HSD2) can cause AME. 11β-HSD2 catalyses the conversion of cortisol to cortisone (Figure 6) to prevent excessive cortisol cross reactivity with mineralocorticoid receptors (but 11β-HSD2 can become saturated if the cortisol concentration is very high, e.g., Cushing Syndrome). If 11β-HSD2 is deficient, cortisol is not inactivated by conversion to cortisone, resulting in increased mineralocorticoid receptor stimulation. Liquorice poisoning can cause dangerous hypokalaemia by similarly inhibiting 11β-HSD2 (60).
Hypokalaemia in pregnancy
There is a very rare mutation in the mineralocorticoid receptor making it sensitive to other steroids such as progesterone. This can result in a syndrome like Liddle (e.g., low renin activity, low aldosterone, hypokalaemia, hypertension with metabolic alkalosis) (42). Called ‘hypertension exacerbated by pregnancy’, this is distinct from other causes of hypertension in pregnancy like pre-eclampsia, and may also present with hypokalaemia (61). Persistent vomiting during pregnancy can also cause hypokalaemia (usually with a metabolic alkalosis).
Conclusions
The investigation of hyperkalaemia and hypokalaemia can be challenging. As well as multiple factors that can cause results that are fake or spurious and not representative of the true in vivo potassium status, many conditions can cause variable potassium levels depending on a host of factors. Here, we present diagnostic algorithms that we hope will help the reader to systematically consider causes of potassium disturbances in a logical order. However, we appreciate that each patient case is different, and it may be appropriate to skip ahead to steps later in the algorithms, refer to other algorithms (e.g., acid base within this series), or even miss out steps entirely. These algorithms cannot replace specialist knowledge, experience and local guidelines. Instead, they should act as a diagnostic aid when assessing patients with potassium disturbances.
Acknowledgments
We would like to thank Professor Rousseau Gama for inspiring and inviting us to prepare this paper. All figures were created with BioRender.com.
Funding: None.
Footnote
Provenance and Peer Review: This article was commissioned by the editorial office, Journal of Laboratory and Precision Medicine for the series “Investigative Algorithms in Laboratory Medicine – Electrolytes and Acid/Base”. The article has undergone external peer review.
Reporting Checklist: The authors have completed the Narrative Review reporting checklist. Available at https://jlpm.amegroups.com/article/view/10.21037/jlpm-22-10/rc
Peer Review File: Available at https://jlpm.amegroups.com/article/view/10.21037/jlpm-22-10/prf
Conflicts of Interest: All authors have completed the ICMJE uniform disclosure form (available at https://jlpm.amegroups.com/article/view/10.21037/jlpm-22-10/coif). The series “Investigative Algorithms in Laboratory Medicine – Electrolytes and Acid/Base” was commissioned by the editorial office without any funding or sponsorship. KS served as the unpaid Guest Editor of the series. The authors have no other conflicts of interest to declare.
Ethical Statement: The authors are accountable for all aspects of the work in ensuring that questions related to the accuracy or integrity of any part of the work are appropriately investigated and resolved.
Open Access Statement: This is an Open Access article distributed in accordance with the Creative Commons Attribution-NonCommercial-NoDerivs 4.0 International License (CC BY-NC-ND 4.0), which permits the non-commercial replication and distribution of the article with the strict proviso that no changes or edits are made and the original work is properly cited (including links to both the formal publication through the relevant DOI and the license). See: https://creativecommons.org/licenses/by-nc-nd/4.0/.
References
- Lehnhardt A, Kemper MJ. Pathogenesis, diagnosis and management of hyperkalemia. Pediatr Nephrol 2011;26:377-84. [Crossref] [PubMed]
- Palmer BF, Clegg DJ. Physiology and Pathophysiology of Potassium Homeostasis: Core Curriculum 2019. Am J Kidney Dis 2019;74:682-95. [Crossref] [PubMed]
- Gumz ML, Rabinowitz L, Wingo CS. An Integrated View of Potassium Homeostasis. N Engl J Med 2015;373:60-72. [Crossref] [PubMed]
- Aronson PS, Giebisch G. Effects of pH on potassium: new explanations for old observations. J Am Soc Nephrol 2011;22:1981-9. [Crossref] [PubMed]
- Youn JH, McDonough AA. Recent advances in understanding integrative control of potassium homeostasis. Annu Rev Physiol 2009;71:381-401. [Crossref] [PubMed]
- Zacchia M, Abategiovanni ML, Stratigis S, et al. Potassium: From Physiology to Clinical Implications. Kidney Dis (Basel) 2016;2:72-9. [Crossref] [PubMed]
- Palmer BF. Regulation of Potassium Homeostasis. Clin J Am Soc Nephrol 2015;10:1050-60. [Crossref] [PubMed]
- Booth RE, Johnson JP, Stockand JD. Aldosterone. Adv Physiol Educ 2002;26:8-20. [Crossref] [PubMed]
- Alfonzo AV, Isles C, Geddes C, et al. Potassium disorders--clinical spectrum and emergency management. Resuscitation 2006;70:10-25. [Crossref] [PubMed]
- Fleet JL, Shariff SZ, Gandhi S, et al. Validity of the International Classification of Diseases 10th revision code for hyperkalaemia in elderly patients at presentation to an emergency department and at hospital admission. BMJ Open 2012;2:e002011. [Crossref] [PubMed]
- Sarafidis PA, Blacklock R, Wood E, et al. Prevalence and factors associated with hyperkalemia in predialysis patients followed in a low-clearance clinic. Clin J Am Soc Nephrol 2012;7:1234-41. [Crossref] [PubMed]
- Morris TG, Lamba S, Fitzgerald T, et al. The potential role of the eGFR in differentiating between true and pseudohyperkalaemia. Ann Clin Biochem 2020;57:444-55. [Crossref] [PubMed]
- Nijsten MW, de Smet BJ, Dofferhoff AS. Pseudohyperkalemia and platelet counts. N Engl J Med 1991;325:1107. [Crossref] [PubMed]
- Asirvatham JR, Moses V, Bjornson L. Errors in potassium measurement: a laboratory perspective for the clinician. N Am J Med Sci 2013;5:255-9. [Crossref] [PubMed]
- Sevastos N, Theodossiades G, Archimandritis AJ. Pseudohyperkalemia in serum: a new insight into an old phenomenon. Clin Med Res 2008;6:30-2. [Crossref] [PubMed]
- Duhalde H, Skogö J, Karlsson M. Point-of-care hemolysis detection in blood gas specimens directly at the emergency department. Scand J Clin Lab Invest 2019;79:283-7. [Crossref] [PubMed]
- Webster A, Brady W, Morris F. Recognising signs of danger: ECG changes resulting from an abnormal serum potassium concentration. Emerg Med J 2002;19:74-7. [Crossref] [PubMed]
- Ben Salem C, Badreddine A, Fathallah N, et al. Drug-induced hyperkalemia. Drug Saf 2014;37:677-92. [Crossref] [PubMed]
- Kidney Disease: Improving Global Outcomes (KDIGO) Work Group. KDIGO Clincal Practice Guideline for Acute Kidney Injury. Kidney Int Suppl 2012;2:1-138.
- Kidney Disease: Improving Global Outcomes (KDIGO) Work Group. KDIGO 2012 Clinical Practice Guideline for the Evaluation and Management of Chronic Kidney Disease. Kidney Int Suppl 2013;3:1-150.
- Newman DJ, Cystatin C. Ann Clin Biochem 2002;39:89-104. [Crossref] [PubMed]
- Marques-Garcia F. Methods for Hemolysis Interference Study in Laboratory Medicine - A Critical Review. EJIFCC 2020;31:85-97. [PubMed]
- Shapira Y, Vaturi M, Sagie A. Hemolysis associated with prosthetic heart valves: a review. Cardiol Rev 2009;17:121-4. [Crossref] [PubMed]
- Lippi G, Blanckaert N, Bonini P, et al. Haemolysis: an overview of the leading cause of unsuitable specimens in clinical laboratories. Clin Chem Lab Med 2008;46:764-72. [Crossref] [PubMed]
- de Jonge G, Dos Santos TL, Cruz BR, et al. Interference of in vitro hemolysis complete blood count. J Clin Lab Anal 2018;32:e22396. [Crossref] [PubMed]
- Shih AW, McFarlane A, Verhovsek M. Haptoglobin testing in hemolysis: measurement and interpretation. Am J Hematol 2014;89:443-7. [Crossref] [PubMed]
- Zimmerman JL, Shen MC. Rhabdomyolysis. Chest 2013;144:1058-65. [Crossref] [PubMed]
- Schifman RB, Luevano DR. Value and Use of Urinalysis for Myoglobinuria. Arch Pathol Lab Med 2019;143:1378-81. [Crossref] [PubMed]
- Gupta A, Moore JA. Tumor Lysis Syndrome. JAMA Oncol 2018;4:895. [Crossref] [PubMed]
- Leighton E, Sainsbury CA, Jones GC. A Practical Review of C-Peptide Testing in Diabetes. Diabetes Ther 2017;8:475-87. [Crossref] [PubMed]
- Kamel KS, Ethier JH, Richardson RM, et al. Urine electrolytes and osmolality: when and how to use them. Am J Nephrol 1990;10:89-102. [Crossref] [PubMed]
- Choi MJ, Ziyadeh FN. The utility of the transtubular potassium gradient in the evaluation of hyperkalemia. J Am Soc Nephrol 2008;19:424-6. [Crossref] [PubMed]
- Bollag WB. Regulation of aldosterone synthesis and secretion. Compr Physiol 2014;4:1017-55. [Crossref] [PubMed]
- Chan S, Debono M. Replication of cortisol circadian rhythm: new advances in hydrocortisone replacement therapy. Ther Adv Endocrinol Metab 2010;1:129-38. [Crossref] [PubMed]
- Martin-Grace J, Dineen R, Sherlock M, et al. Adrenal insufficiency: Physiology, clinical presentation and diagnostic challenges. Clin Chim Acta 2020;505:78-91. [Crossref] [PubMed]
- Han TS, Walker BR, Arlt W, et al. Treatment and health outcomes in adults with congenital adrenal hyperplasia. Nat Rev Endocrinol 2014;10:115-24. [Crossref] [PubMed]
- Marques P, Tufton N, Bhattacharya S, et al. Hypertension due to a deoxycorticosterone-secreting adrenal tumour diagnosed during pregnancy. Endocrinol Diabetes Metab Case Rep 2019;2019:18-0164. [Crossref] [PubMed]
- El-Maouche D, Arlt W, Merke DP. Congenital adrenal hyperplasia. Lancet 2017;390:2194-210. [Crossref] [PubMed]
- Rutledge AC, Johnston A, Bailey D, et al. Survey of renin and aldosterone testing practices by Ontario laboratories - Providing insight into best practices. Pract Lab Med 2021;25:e00229. [Crossref] [PubMed]
- Weber F, Adam MPMirzaa GM, et al. Hyperkalemic Periodic Paralysis 1993.
- Amin N, Alvi NS, Barth JH, et al. Pseudohypoaldosteronism type 1: clinical features and management in infancy. Endocrinol Diabetes Metab Case Rep 2013;2013:130010. [Crossref] [PubMed]
- Enslow BT, Stockand JD, Berman JM. Liddle's syndrome mechanisms, diagnosis and management. Integr Blood Press Control 2019;12:13-22. [Crossref] [PubMed]
- Kardalas E, Paschou SA, Anagnostis P, et al. Hypokalemia: a clinical update. Endocr Connect 2018;7:R135-46. [Crossref] [PubMed]
- Sodi R, Davison AS, Holmes E, et al. The phenomenon of seasonal pseudohypokalemia: effects of ambient temperature, plasma glucose and role for sodium-potassium-exchanging-ATPase. Clin Biochem 2009;42:813-8. [Crossref] [PubMed]
- Polak R, Huisman A, Sikma MA, et al. Spurious hypokalaemia and hypophosphataemia due to extreme hyperleukocytosis in a patient with a haematological malignancy. Ann Clin Biochem 2010;47:179-81. [Crossref] [PubMed]
- Veltri KT, Mason C. Medication-induced hypokalemia. P T 2015;40:185-90. [PubMed]
- Mehanna HM, Moledina J, Travis J. Refeeding syndrome: what it is, and how to prevent and treat it. BMJ 2008;336:1495-8. [Crossref] [PubMed]
- Neumann HPH, Young WF Jr, Eng C. Pheochromocytoma and Paraganglioma. N Engl J Med 2019;381:552-65. [Crossref] [PubMed]
- Lenders JW, Pacak K, Walther MM, et al. Biochemical diagnosis of pheochromocytoma: which test is best? JAMA 2002;287:1427-34. [Crossref] [PubMed]
- Carmel R. How I treat cobalamin (vitamin B12) deficiency. Blood 2008;112:2214-21. [Crossref] [PubMed]
- Arasaradnam RP, Brown S, Forbes A, et al. Guidelines for the investigation of chronic diarrhoea in adults: British Society of Gastroenterology, 3rd edition. Gut 2018;67:1380-99.
- Whang R, Whang DD, Ryan MP. Refractory potassium repletion. A consequence of magnesium deficiency. Arch Intern Med 1992;152:40-5. [Crossref] [PubMed]
- Huang CL, Kuo E. Mechanism of hypokalemia in magnesium deficiency. J Am Soc Nephrol 2007;18:2649-52. [Crossref] [PubMed]
- Yang L, Frindt G, Palmer LG. Magnesium modulates ROMK channel-mediated potassium secretion. J Am Soc Nephrol 2010;21:2109-16. [Crossref] [PubMed]
- Davis SM, Maddux AB, Alonso GT, et al. Profound hypokalemia associated with severe diabetic ketoacidosis. Pediatr Diabetes 2016;17:61-5. [Crossref] [PubMed]
- Alexandridis G, Elisaf M. Renal potassium handling in a patient with surreptitious vomiting. Nephrol Dial Transplant 2001;16:2275. [Crossref] [PubMed]
- Schirpenbach C, Reincke M. Primary aldosteronism: current knowledge and controversies in Conn's syndrome. Nat Clin Pract Endocrinol Metab 2007;3:220-7. [Crossref] [PubMed]
- Vogg N, Kurlbaum M, Deutschbein T, et al. Method-Specific Cortisol and Dexamethasone Thresholds Increase Clinical Specificity of the Dexamethasone Suppression Test for Cushing Syndrome. Clin Chem 2021;67:998-1007. [Crossref] [PubMed]
- Phuyal P, Nagalli S. Hypokalemic Periodic Paralysis. In: Treasure Island (FL); 2021.
- Edelman ER, Butala NM, Avery LL, et al. Case 30-2020: A 54-Year-Old Man with Sudden Cardiac Arrest. N Engl J Med 2020;383:1263-75. [Crossref] [PubMed]
- Pintavorn P, Munie S. A Case Report of Recurrent Hypokalemia During Pregnancies Associated With Nonaldosterone-Mediated Renal Potassium Loss. Can J Kidney Health Dis 2021;8:20543581211017424. [Crossref] [PubMed]
Cite this article as: Flowers KC, Allen GT, Darragh-Hickey C, Kaur S, Shipman AR, Shipman KE. Investigative algorithms for disorders affecting plasma potassium: a narrative review. J Lab Precis Med 2022;7:25.