Investigative algorithms for disorders affecting plasma sodium: a narrative review
Introduction
Sodium is the major extracellular cation in humans and is the largest contributor to plasma osmolality (1). Sodium is essential for two major processes in the body; fluid balance (and therefore blood pressure) and nervous excitation. Although only approximately 5% of total body sodium resides intracellularly at a concentration between 10–20 mmol/L, only 10% of total body sodium is located in the plasma at a concentration between 135–145 mmol/L (1). Approximately 45% of total body sodium is located in bone, skin and muscle, 10% is located in connective tissue, and 30% is located in the interstitial fluid (Figure 1) (1).
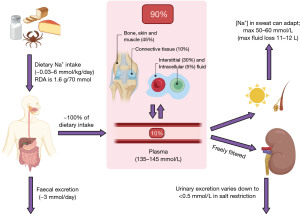
Plasma sodium analysis can be a useful tool for assessing patients who are hyponatraemic or hypernatraemic. However, sodium analysis is prone to providing results that are not an accurate reflection of the true in vivo status, and being aware of this is imperative for appropriate patient management. The following algorithms aim to provide a rough guide on using laboratory diagnostics to evaluate the causes of sodium disturbances; particularly for those with less experience of patient assessment where a recommended sequence of steps can help to ensure good quality care and use of diagnostic resources.
An in depth review of sodium homeostasis is beyond the scope of this algorithm; many detailed reviews have been published elsewhere (1-6). However, for context, Figure 2 demonstrates a summary of renal sodium handling. Sodium is freely filtered at the glomerulus, and 50–60% is reabsorbed in the proximal convoluted tubule (PCT), 30–40% is reabsorbed in the thick ascending limb (TAL) and 5–10% is reabsorbed in the distal convoluted tubule (DCT) (4).
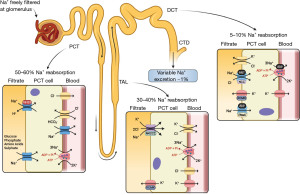
In the DCT and collecting duct, the hormone aldosterone acts on sodium chloride cotransporters (NCC), epithelial sodium channels (ENaC) and proton uniporter channels on the apical (filtrate) membrane, and sodium-potassium pumps on the basolateral (blood) membrane, to increase sodium (and therefore water) reabsorption from the filtrate (Figure 2) (7). Aldosterone release is primarily controlled via the renin angiotensin aldosterone system (RAAS). Firstly, decreased plasma volume is detected by the juxtaglomerular cells in the kidney, which release renin. Renin converts angiotensinogen to angiotensin I, which is then converted to angiotensin II in the lungs by angiotensin converting enzyme (ACE) (8). Angiotensin II has multiple actions, but its primary action is to stimulate aldosterone secretion from the adrenal glands (Figure 3) (8). The RAAS is a significant mechanism of plasma volume control through its direct influence on sodium reabsorption, which in turn influences water reabsorption.
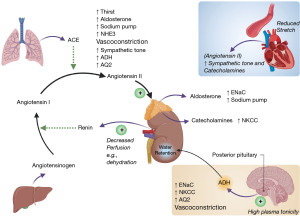
Anti-diuretic hormone (ADH), also commonly referred to as arginine vasopressin, is released from the posterior pituitary gland when an increase in plasma tonicity is detected by osmoreceptors in the hypothalamus (6). ADH is also released in response to decreased plasma volume via direct stimulation from angiotensin II (8). ADH acts on the renal collecting ducts to increase expression of aquaporin II channels, leading to increased passive free water reabsorption and more concentrated urine (5,9). Although these mechanisms are complex in reality, a useful way to view aldosterone is it primarily acts to increase sodium reabsorption from the renal filtrate (which then causes water reabsorption), whereas ADH primarily acts to increase free water reabsorption. Figure 3 shows a basic overview of the RAAS and ADH secretion and action. We present the following article in accordance with the Narrative Review reporting checklist (available at https://jlpm.amegroups.com/article/view/10.21037/jlpm-22-11/rc).
Methods
The narrative literature review was created by searching Medline, Google Scholar, OMIM and seminal texts. The diagnostic algorithms were then created using the information gathered for the literature review. The literature was searched over the period September 2021 to January 2021. The language was restricted to English. For further information please see Supplementary file (Table S1).
Hyponatraemia
Hyponatraemia is defined as a serum sodium of <135 mmol/L, and it is the most commonly encountered electrolyte disorder in clinical practice with a reported incidence of 15–30% (10). Hyponatraemia is often categorised as mild (130–134 mmol/L), moderate (126–130 mmol/L) or severe (<125 mmol/L) (10). It can be symptomatic or asymptomatic, and this largely depends on the level to which the sodium has fallen and the rate of the decrease. Unless serum sodium has fallen rapidly, concentrations in the range 125–135 mmol/L are often asymptomatic. There are a number of causes of hyponatraemia and, especially in older patients, it is likely to be multi-factorial. Here, we present an algorithm for the laboratory investigation of hyponatraemia (Figure 4).
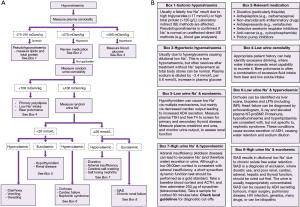
Plasma osmolality
A very useful test in the assessment of hyponatraemia is plasma osmolality. Osmolality is a measure of the osmotically active solute particles dissolved per kg of solvent (e.g., water when measuring plasma), as opposed to osmolarity which is a measure of osmotically active solute particles dissolved per litre of solvent. Although technically different, distinguishing osmolality from osmolarity makes very little difference clinically, so the terms are often used interchangeably.
Two of the most common methods for measuring plasma osmolality include freezing point depression and vapour pressure. However, freezing point depression is the recommended method as vapour pressure can fail to detect some osmotically active volatile substances (11). Multiple equations to calculate the expected osmolality have been proposed, but a simple and easy to implement equation is displayed below. All units are in mmol/L, and the sodium concentration is multiplied by two to account for the accompanying anions that maintain electroneutrality (e.g., Cl− and HCO3−). As calculated osmolality equations use concentrations of analytes per litre of solution (e.g., sodium 145 mmol/L) and not per kg of solution, calculated osmolality is technically calculated osmolarity. However, for simplicity, this will be referred to as calculated osmolality.
Comparison of the calculated and measured osmolality is a useful first step when investigating hyponatraemia. As sodium is the major component of plasma osmolality, a low plasma sodium (and therefore a low calculated osmolality) should be accompanied by a proportionately low measured osmolality (<275 mOsm/kg), which is termed hypotonic hyponatraemia. A normal measured osmolality (275–295 mOsm/kg) during hyponatraemia, which is termed isotonic hyponatraemia, suggests that the sodium result may not be correct. A high measured plasma osmolality (>295 mOsm/kg) during hyponatraemia is termed hypertonic hyponatraemia, and this means that another major osmotically active substance is present.
Isotonic hyponatraemia (including pseudohyponatraemia)
A normal osmolality during hyponatraemia is most likely due to pseudohyponatraemia caused by a significantly elevated total protein (>120 g/L) or triglyceride (>17 mmol/L) concentration (12). Grossly elevated cholesterol concentrations (>35 mmol/L) in the context of cholestasis (e.g., primary biliary cholangitis) can also be the cause due to accumulation of lipoprotein X (13,14).
Only indirect ion selective electrode (ISE) sodium methods used in central laboratories produce pseudohyponatraemia due to these causes; direct ISE sodium methods used in blood gas analysers are not affected. Understanding this important difference requires appreciating that on average, total plasma is composed of 93% water and 7% solid phase (e.g., proteins and lipids) (12). Sodium cannot reside in the solid phase, so the concentration of sodium in the water phase is the physiologically important value. Indirect ISE methods are indirect because they dilute a fixed volume of total plasma before analysis (in order to use less plasma and to increase the top end of the reportable range). As sodium can only reside in the water phase of the total plasma volume, sodium cannot exist in 100% of the total plasma volume that is aliquoted for the test. In order to take this into account, it is assumed that the water phase occupies 93% of the total plasma volume in all patients. To do this, the total plasma volume aliquoted for the test is essentially multiplied by 0.93 before the measured sodium result is multiplied by the dilution factor (approximately ×30). When the solid phase of plasma is increased—which is the case in hyperproteinaemia >120 g/L (e.g., multiple myeloma or intravenous immunoglobulin administration), hypertriglyceridaemia >17 mmol/L (12) or severe hypercholesterolaemia (14)—this assumption is no longer accurate. In these cases, although the sodium concentration in the water phase remains the same, the same volume of total plasma that is aliquoted for the test actually contains less water (e.g., 84%), and by extension, less sodium, leading to underestimation of the final sodium concentration (Figure 5). This also occurs with other ions measured by indirect ISE (e.g., potassium and chloride), but is usually less obvious as they are present in smaller concentrations than sodium.
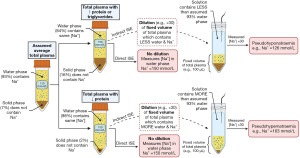
Direct ISE methods in blood gas analysers directly measure the sodium activity in the clinically relevant water phase without dilution, therefore the sodium result is much more reliable (when the plasma water phase is not 93%) than indirect methods (15). If osmolality measurement is not available, a higher sodium result using a direct ISE blood gas analyser than a sodium result using an indirect ISE laboratory method strongly suggests that pseudohyponatraemia is occurring.
Pseudohyponatraemia also occurs if the sample is contaminated with fluids which contain less sodium than normal plasma (e.g., dextrose). This should be considered if many analytes (including sodium) are low or have decreased rapidly since the previous result. Albumin is particularly useful in this context (if a recent previous result is available) as it has a half-life of approximately 21 days (16), therefore a significant decrease in a shorter timeframe may be suspicious. Dextrose contamination can be avoided by always taking blood samples from the opposite arm from the infusion, or stopping infusions at least five minutes prior to venesection.
It is important to note that isotonic hyponatraemia does not always mean pseudohyponatraemia, and can also be a result of the timing of plasma osmolality analysis in situations where a true hypertonic hyponatraemia is usually expected. For example, it is possible that there is the just the right amount of ethanol in plasma [as ethanol is metabolised rapidly (17)], that in conjunction with hyponatraemia, can make the measured osmolality normal instead of high. This should be suspected if the total protein and triglyceride concentrations are normal, or the direct ISE sodium result is similarly low to the indirect ISE sodium result, during isotonic hyponatraemia.
Hypertonic hyponatraemia
This is most commonly caused by hyperglycaemia (5), but can also be caused by mannitol, ethanol, methanol or ethylene glycol (before the toxic alcohols are metabolised). Glucose and mannitol are ‘effective solutes’ because they remain in the extracellular compartment. Therefore, they contribute to plasma tonicity, attract water from the intracellular compartment and can directly cause dilutional hyponatraemia. Importantly however, although ethanol and methanol contribute to measured osmolality, they are ‘ineffective solutes’ because they freely cross cell membranes into the intracellular compartment. Therefore, they do not contribute to plasma tonicity, do not attract water from the intracellular compartment and do not directly cause hyponatraemia (11).
Hyponatraemia from hyperglycaemia is often incorrectly referred to as pseudohyponatraemia. Importantly, this is actually a true dilutional hyponatraemia (5), and incorrectly referring to this as spurious may not highlight the risk of cerebral oedema if the glucose concentration is reduced too quickly (10). This confusion may arise because the total body sodium is often normal in these cases (before osmotic diuresis occurs), and simply treating the hyperglycaemia frequently corrects the hyponatraemia without any sodium replacement. For every 5.6 mmol/L increase in glucose concentration, plasma sodium is truly diluted and decreases by approximately 2.4 mmol/L (18).
More formally, the osmolar gap can be calculated by simply taking the calculated osmolality away from the measured osmolality. An osmolar gap >10 mOsm/kg suggests that there is another osmotically active substance present in the plasma that is not accounted for during the calculation. Calculating the osmolar gap can be useful to help in determining what substance is contributing to a high osmolar gap and the extent of the effect. For example, alcohol can be included as below (all units in mmol/L) (19).
Hypotonic hyponatraemia
Hypotonicity is the most common tonicity finding in hyponatraemia, and reflects a true hyponatraemia in the absence of other osmotically active substances. A quick next step is to review the medications (20) that the patient is taking to see if any are known to cause hyponatraemia (Figure 4B). If the patient is taking any medications known to cause hyponatraemia, it may be appropriate to stop them or to reduce the dose, and then periodically monitor plasma sodium.
Random urine osmolality
The next most useful step is to test osmolality on a random urine sample. A urine osmolality <100 mOsm/kg demonstrates that the urine is maximally dilute. Normally, hypotonic fluid intake is balanced by decreasing ADH secretion in order to increase free water excretion, leading to dilution of the urine and maintenance of the plasma sodium concentration. However, excessive free water intake can exceed the kidney’s maximum capability to excrete it, leading to dilution of sodium in the plasma (5). Less commonly, maximally dilute urine could also be due to low sodium intake. In people who misuse alcohol, particularly people who drink excessive amounts of relatively high water to alcohol ratio beverages (e.g., beer and cider), hyponatraemia can be caused by a combination of excess water ingestion and insufficient sodium intake (termed beer potomania) (21). A similar manifestation can also occur in marathon runners after a race (22), where they drink excessive volumes of hypotonic fluids [either pure water or sports drinks that only contain ~18 mmol/L of sodium (23)] in a short period of time, and lose sodium via sweat.
Random urine sodium
During hyponatraemia, a random urine sodium <20 mmol/L suggests that the kidneys are appropriately reabsorbing sodium from the renal filtrate, and that extrarenal sodium loss or dilution is occurring. A random urine sodium ≥20 mmol/L suggests that the kidneys are failing to appropriately reabsorb sodium from the filtrate, and sodium is being inappropriately excreted in the urine. Following the algorithm in Figure 4A, the urine sodium concentration can be used in conjunction with a clinical assessment of the volume status to further narrow down the likely cause of hyponatraemia.
Fluid status
Hyponatraemia can be caused by loss of total body sodium, or more commonly, total body sodium dilution from water retention (impaired water excretion). Determining the fluid status of the patient is important to help determine which one of these mechanisms is occurring, which will significantly narrow down the likely diagnoses. However, determining the fluid status can often be difficult in reality and can lead to misclassification (24), so it is perhaps appropriate to include this assessment later in the algorithm than easier to perform investigations (although some cases can be very obvious and hence quickly identified). A full description of how to perform a fluid examination is beyond the scope of this article, but excellent reviews of this topic have been published elsewhere (25,26).
Hypovolaemic hyponatraemia
If a patient is hypovolaemic and the kidneys are appropriately reabsorbing sodium from the filtrate (random urine sodium <20 mmol/L), it is likely that extrarenal sodium loss is occurring (as water will follow sodium to decrease total body fluid). This can be caused by diarrhoea, vomiting and/or excessive sweating, and an appropriate patient history is key to identifying these (27). Diarrhoea and vomiting may also be accompanied by potassium, chloride and acid base disturbances (refer to the relevant articles within this series). Guidelines for the management of patients with diarrhoea have been published elsewhere (27).
If a patient is hypovolaemic and the kidneys are inappropriately excreting sodium (random urine sodium >20 mmol/L), it is likely that there is an issue either directly or indirectly that is preventing the kidneys from reabsorbing sodium. The most common cause of this is diuretic use, which decrease sodium reabsorption from the filtrate leading to salt and water loss. Adrenal insufficiency can also cause renal sodium loss and can be a medical emergency.
Adrenal insufficiency in hypovolaemic hyponatraemia
As aldosterone is a significant stimulator of sodium reabsorption from the filtrate (Figure 2), adrenal insufficiency (aldosterone and cortisol deficiency) can lead to sodium wasting in the urine and hypovolaemia. This is usually a medical emergency and requires immediate treatment. Primary adrenal insufficiency (Addison disease) should be particularly suspected if there is a concurrent hyperkalaemia. However, absence of hyperkalaemia does not rule out adrenal insufficiency, particularly secondary adrenal insufficiency where normokalaemia is common (see potassium article within this series).
Adrenal function can be assessed by the ability to secrete cortisol. Cortisol exhibits a diurnal variation, where it is highest in the morning and lowest in the evening (28). A blood cortisol concentration less than a defined cut-off (check local guidelines) on a sample taken at around 09:00 am may be inappropriately low, suggesting that the adrenal gland is not functioning correctly (Addison disease). A 09:00 am cortisol above a specific local cut-off virtually excludes Addison disease (29).
The gold standard for confirming adrenal insufficiency is a short synacthen (synthetic ACTH) test (SST), which directly stimulates the adrenal gland to produce cortisol to assess if the response is adequate (29). The procedure for performing an SST is described in Figure 4B. As cortisol cut-offs are dependent on the cortisol method a laboratory uses and the local population, it is important to always check local guidelines to avoid misdiagnosis.
There are important caveats to consider when interpreting SST results. For example, long term secondary adrenal insufficiency, and prolonged exogenous steroid replacement/abuse, can give SST results similar to primary adrenal insufficiency due to adrenal atrophy. Use of oestrogen (e.g., contraceptive pill or hormone replacement therapy) will increase the cortisol binding globulin concentration (29). This will therefore increase total cortisol, giving spuriously normal cortisol measurements in deficiency states (hormones need stopping six weeks prior to cortisol analysis). The risk of false negatives is concerning.
Adrenal insufficiency can be caused by tuberculosis, human immunodeficiency virus (HIV), autoimmunity, physical trauma, drugs, haemorrhage, or congenital adrenal hyperplasia (CAH) (30). CAH is characterised by a genetic deficiency of an enzyme that is involved in steroidogenesis (including aldosterone and cortisol synthesis) in the adrenal glands (31). There are many mutations which can lead to hyponatraemia causing enzyme deficiencies with various phenotypes, but 21-hydroxylase deficiency accounts for more than 95% of cases (31). In 21-hydroxylase deficiency, there is both impaired aldosterone and cortisol synthesis, leading to hyponatraemia, hyperkalaemia, hypovolaemia and virilisation in females (due to pathway shunting to increase testosterone production). Figure 6 shows the adrenal steroidogenesis pathway and many of the other enzymes that can be affected in CAH (31,32). It is important to note that 11-deoxycorticosterone possesses weak mineralocorticoid activity, so enzyme deficiencies that significantly increase its level (e.g., 11β-hydroxylase and 17α-hydroxylase) can conversely cause hypernatraemia, hypertension and hypokalaemia (31). The diagnosis and treatment of CAH can vary depending on the enzyme defect. El-Maouche et al. (31) have published a detailed review of CAH if more information is required.
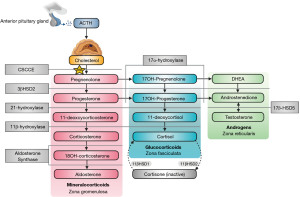
Other causes of hypovolaemic hyponatraemia
Cerebral salt wasting (CSW) is a cause of hyponatraemia that can be seen in patients with central nervous system (CNS) abnormalities, particularly after subarachnoid haemorrhage (33). The mechanism of CSW is poorly understood, but it is believed to be due to a mixture of disturbance of CNS regulation of salt and volume homeostasis, and the presence of circulating brain natriuretic peptide (33). Biochemical presentations are similar to the syndrome of inappropriate antidiuresis (SIAD), but the key difference is the presence of hypovolaemia in conjunction with an appropriate patient history (33).
Salt losing nephritis (SLN) is essentially the wasting of sodium chloride in the kidneys. SLN can present very similarly to Addison disease, but key differences include normal adrenal function and the presence of renal disease (34).
Euvolaemic hyponatraemia
SIAD, also known as syndrome of inappropriate antidiuretic hormone (SIADH), is a common cause of euvolaemic hyponatraemia with a random urine sodium ≥20 mmol/L (due to poor ability to dilute urine) (35). SIAD causes hyponatraemia due to inappropriate increased concentration of ADH, which leads to excessive free water reabsorption from the renal filtrate, dilution of plasma sodium and the inappropriate concentration of urine (urine osmolality ≥100 mOsm/kg). Although there is slight expansion in blood volume and subsequent suppression of renin, this does not manifest clinically as hypervolaemia (35). There are many causes of SIAD including drugs (e.g., carbamazepine, selective serotonin reuptake inhibitors), surgery, pulmonary disease, HIV infection, genetic, ADH secreting tumours and idiopathic (36). Table 1 summarises the diagnostic criteria for SIAD (35). Importantly, SIAD is a diagnosis of exclusion, where other causes of hyponatraemia including adrenal insufficiency, hypothyroidism, cardiac disease, liver disease and renal disease have been ruled out first.
Table 1
Plasma sodium <135 mmol/L |
Plasma osmolality <275 mOsm/kg |
Random urine sodium >20 mmol/L |
Random urine osmolality >100 mOsm/kg |
Clinically euvolaemic |
Other causes of hyponatraemia (e.g., cardiac, hepatic, renal, adrenal, thyroid disease) ruled out |
If the patient is appropriately reabsorbing urine from the renal filtrate (random urine sodium <20 mmol/L), hypothyroidism should be ruled out by analysing plasma thyroid stimulating hormone (TSH) and free-T4 [although hypothyroidism is considered to be a rare cause of hyponatraemia (5)]. Adrenal insufficiency is excluded as described previously. If acute or chronic renal disease is suspected, the quickest and simplest method to assess renal function is to measure the serum/plasma creatinine concentration and monitor the urine output (37,38).
Hypervolaemic hyponatraemia
If a patient is fluid overloaded during hyponatraemia, the hyponatraemia is primarily due to plasma sodium dilution with free water. Excess free water retention can result from impaired excretion and/or inappropriate reabsorption from the renal filtrate. This usually requires treatment with diuretics to help excrete excess fluid. Although hyponatraemic, saline should not be given to hypervolaemic patients as they are often not total body sodium deplete, and excess sodium can freely cross into the extracellular fluid which can exacerbate the hypervolaemia.
Cirrhosis causes hyponatraemia by various mechanisms, and John and Thuluvath have published a good review of this pathogenesis (39). Cirrhosis can be diagnosed by abnormal liver function test (LFT) results, or the observation of scarring on liver ultrasound or biopsy. However, LFTs do not become abnormal until cirrhosis is advanced. More sensitive blood biomarker methods including the Fibrosis 4 (40) and Enhanced Liver Fibrosis (ELF™) (41) scores have been proposed.
Hyponatraemia in cardiac failure is mainly triggered by retention of sodium and water by the kidneys (42). Diagnosing cardiac failure can be done by a combination of clinical examination, x-ray, echocardiography and blood tests (43). A useful blood test is brain natriuretic peptide (BNP) or NT-proBNP, which are released in response to left ventricular wall stretching seen in cardiac failure. Although issues such as concurrent renal impairment can independently increase the concentration of these analytes in plasma and complicate their interpretation, a concentration of either below a specific cut-off (check local guidelines) rules out cardiac failure due to their very high negative predictive value (43).
Nephrotic syndrome is characterised by a protein excretion in urine of >3.5 g/24 h (or a random urine protein:creatinine ratio >350 mg/mmol), plasma albumin concentration <25 g/L, peripheral oedema and hyperlipidaemia (total cholesterol is often >10 mmol/L) (44). Nephrotic syndrome causes renal albumin wasting, which decreases the plasma albumin concentration and results in lower plasma oncotic pressure. This leads to low plasma volume which triggers the RAAS, causing sodium and water retention manifesting as oedema. As anti-thrombotic factors are also wasted renally, these patients are at increased risk of thrombosis (44).
Rare causes of hyponatraemia
Although aldosterone acts to reduce sodium excretion, isolated aldosterone deficiency very rarely causes hyponatraemia due to other compensatory mechanisms (e.g., cortisol mineralocorticoid receptor cross reactivity and cortisol inhibition of ADH, and the effects of angiotensin II and noradrenaline) (45). However, cases of this nature have been reported in the literature (46). Aldosterone and renin activity measurement may be useful to help diagnose these rare cases.
Acute neurovisceral attacks in porphyria are a rare but recognised cause of hyponatraemia due to SIAD (47). If acute porphyria is suspected, analysis of total porphyrins, porphobilinogen and aminolaevulinic acid in a random urine sample (and blood in some cases) after symptoms have manifested is appropriate for diagnosis. Importantly, the urine can remain positive for porphyrin precursors for as long as 20 years after an acute attack has occurred, therefore immediate urine analysis is not necessarily required in order to make the diagnosis (48).
Total body sodium deficiency in eunatraemia
A person can be salt and water deplete even whilst eunatraemic. Two of the main tests that are commonly used to determine if sodium replacement is required are random urine sodium or plasma bicarbonate. In response to hypovolaemia from depleting total body sodium, aldosterone causes sodium reabsorption in the renal tubules at the expense of potassium and hydrogen ions (Figure 2). Therefore, a ‘contraction alkalosis’ develops, possibly with hypokalaemia which can be more prevalent depending on the cause of the fluid loss (e.g., more common in post gastro-intestinal surgery patients with ileus) (49). If the bicarbonate concentration is high or urine sodium is low, even if normotensive and eunatraemic, more salt and water may be required. Assessment using plasma bicarbonate may be more practical if the patient is being bled for other analytes regularly, and is actually preferred as urine sodium can be misleadingly >20 mmol/L in contraction alkalosis during sodium depletion (49).
Hypernatraemia
Hypernatraemia is defined as a sodium concentration >145 mmol/L and represents a deficit of water in relation to the total body stores of sodium (50). This can result from sodium gain, or much more commonly, from a net water loss (including insufficient water intake or excessive free water excretion) (50). As sodium is a major contributor to plasma tonicity, hypernatraemia is a powerful stimulant of thirst and ADH release (Figure 3). Therefore, sustained hypernatraemia can only occur when access to water or the thirst response is impaired. Patients at the highest risk of sustained hypernatraemia include the elderly, where they are often less able to freely consume water due to mental and physical constraints, and have a decreased thirst drive (50). Inpatients are also at risk of hypernatraemia as they are more likely to rely on others for the provision of enteral or parenteral fluids. Symptoms of hypernatraemia primarily reflects CNS dysfunction, and these are more likely to be present if the increase in plasma sodium is rapid (50). Here, we present an algorithm for the laboratory investigation of hypernatraemia (Figure 7).
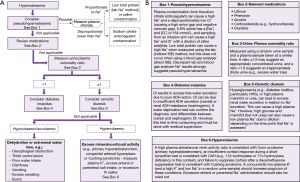
Pseudohypernatraemia
Pseudohypernatraemia should always be considered in every case of hypernatraemia, particularly if the patient is asymptomatic or the result is surprising. Pseudohypernatraemia is not as commonly considered as pseudohyponatraemia, but the clinical risk is just as severe if not detected. Pseudohypernatraemia in inpatients is perhaps most commonly caused by sample contamination with saline, which can happen when the sample is taken from the same arm as a saline infusion. Saline (0.9%) contains 154 mmol/L of sodium and chloride, therefore an elevated sodium and chloride with a low concentration of other analytes should trigger suspicion of saline contamination. Albumin is particularly useful in this context (if a recent previous result is available) as it has a half-life of approximately 21 days (16), therefore a significant decrease in a shorter timeframe may be suspicious.
In the exact opposite mechanism to pseudohyponatraemia, a low total protein can cause pseudonormonatraemia (in patients with a true hyponatraemia) or pseudohypernatraemia when sodium is analysed using an indirect ISE method (Figure 5). Similarly to pseudohyponatraemia, this effect does not occur when sodium is measured using a direct ISE method such as a blood gas analyser. This mechanism of causing pseudohypernatraemia has been described more recently than the mechanism of pseudohyponatraemia (51), and therefore may be under recognised in clinical practice. It has been shown that for every 10 g/L decrease in total protein, plasma sodium results by indirect ISE increase by approximately 0.7 mmol/L (52,53). In 25% of patients admitted to intensive care (where hypoproteinaemia is common), sodium results obtained via indirect ISE are ≥4 mmol/L higher than they would be if obtained via direct ISE (54). Consequently, it has been proposed that only direct ISE methods should be used in patients with a low total protein (52,54).
Pseudohypernatraemia can also be caused by direct contamination from trisodium citrate. Trisodium citrate is commonly found in blood tubes used for coagulation studies, and in Citra-lock™ anticoagulant which is used to ‘lock’ catheters to keep them functional between treatments (55). Sodium and chloride exist in plasma at a ratio of approximately 1.4:1.0, therefore true hypernatraemia is usually accompanied by hyperchloraemia. However, as sodium citrate does not contain chloride and exists in liquid form that can also dilute the blood sample, contamination can cause hypernatraemia with a uncharacteristically low or normal plasma chloride (56,57). As there is only one mole of citrate for every three moles of sodium (both of which are osmotically active) in trisodium citrate blood tubes, the measured osmolality is usually unexpectedly low compared to the calculated osmolality in contamination, and there can even be a negative osmolar gap (57). However, contamination with Citra-lock™ often provides a higher measured osmolality than contamination with trisodium citrate from blood tubes due to the much higher concentration of trisodium citrate (56). Trisodium citrate is also a weak chelator, therefore low concentrations of calcium, magnesium, alkaline phosphatase and zinc can also be observed in contamination (although usually not to the same degree as EDTA contamination).
Medication causes of hypernatraemia
Compared to hyponatraemia, fewer drugs are associated with hypernatraemia. Perhaps the most recognised is lithium therapy which causes nephrogenic diabetes insipidus (DI) (see DI section). Liamis et al. (58) have published a comprehensive review of drug induced hypernatraemia if more information is required.
Urine: plasma osmolality ratio
As hypernatraemia usually represents a total body deficit of water, the normal response is to secrete ADH to stimulate free water reabsorption from the renal filtrate, concentrating the urine (urine:plasma osmolality ratio ≥1) in the process. If the urine:plasma osmolality ratio is <1, this means that the urine is inappropriately dilute, suggesting that there is a failure of free water reabsorption from the filtrate and excess water excretion.
Diabetes insipidus (urine:plasma osmolality ratio <1)
Diabetes insipidus (DI) is characterised by polyuria (>50 mL/kg/24 h) and polydipsia (59), and is caused by a deficiency of ADH (cranial DI) or resistance to its action (nephrogenic DI). Lack of ADH action leads to free water wasting in the kidney, causing an increase in plasma osmolality and extreme thirst. Plasma sodium can be normal as well as high depending on if enough water is being consumed to replace the free water wasting. Clinically, primary polydipsia can present similarly to DI in that patients also present with polyuria. However, the key difference physiologically is that the polydipsia causes the polyuria in primary polydipsia, whereas the polyuria causes the polydipsia in DI (59). Additionally, hyponatraemia makes primary polydipsia more likely and hypernatraemia makes DI more likely, although cases can be more difficult to differentiate as plasma sodium can be normal in both conditions.
The gold standard to diagnose DI is a water deprivation test (WDT). If DI is then determined to be likely, a desmopressin challenge can then be used to help differentiate between cranial and nephrogenic DI (60). Conducting WDTs can be very dangerous if a person does actually have DI, therefore it must be conducted under direct medical supervision and the test aborted if certain safety thresholds are exceeded. Alternatively, copeptin analysis—the C-terminal segment of the ADH prohormone—may have utility as a direct assessment of ADH concentration instead of indirect WDTs (61). Causes and risk factors of DI are summarised in Table 2. Excellent reviews of DI pathogenesis, diagnosis and treatment have been published elsewhere (59,60).
Table 2
Type of DI | Causes |
---|---|
Cranial | Trauma |
Surgery | |
Idiopathic | |
Neoplasia | |
Infection (e.g., meningitis, tuberculosis) | |
Sarcoidosis | |
Haemorrhage, infarction or aneurysm | |
Drugs and toxins | |
Osmoreceptor dysfunction | |
Nephrogenic | Drugs (e.g., lithium, cisplatin) |
Hypercalcaemia | |
Hypokalaemia | |
Amyloidosis and sarcoidosis | |
Sickle cell anaemia | |
Gestational | The placenta produces vasopressinase which can degrade circulating ADH—typically in the second/third trimester |
DI, diabetes insipidus; ADH, antidiuretic hormone.
Osmotic diuresis
Although high glucose and mannitol are associated with dilutional hyponatraemia, they can also cause hypernatraemia via osmotic diuresis at different stages of their metabolism. Excess filtration of osmotically active substances can impair the reabsorption of free water and sodium (and other analytes) (62), potentially causing hypernatraemia if relatively less water can be reabsorbed from the filtrate. High urea can also cause osmotic diuresis, and is a common cause of hypernatraemia in critically ill patients (63).
Fluid status
Hypovolaemic hypernatraemia
Hypovolaemic hypernatraemia suggests that the more common scenario of hypernatraemia, where there is a total body deficiency of water compared to sodium, is likely. This relative water deficiency can be caused by poor water intake or excess water loss. Poor intake is particularly common in individuals with physical or mental constraints. However, poor intake can also occur independently in patients with oesophageal obstructions and thirst centre lesions. Diarrhoea, vomiting, sweating and burns can all lead to relative water loss and cause hypernatraemia. Examination of the patient and an extensive patient history are key to identify causes of poor water intake or loss.
Hypervolaemic hypernatraemia
Hypervolaemic hypernatraemia suggests that both sodium and water retention is occurring. In a hospital setting, this is most likely due to excessive sodium and fluid administration via enteral or parenteral routes. Excess mineralocorticoid activity, which can occur in primary hyperaldosteronism (Conn syndrome), CAH (e.g., 11β-hydroxylase and 17α-hydroxylase deficiency) and hypercortisolism (Cushing disease) can also cause sodium and water retention. A concurrent hypokalaemia should increase suspicion of these conditions, but this biochemical pattern is not specific. See the potassium article within this series for more details regarding the diagnosis of these conditions.
Conclusions
The investigation of hyponatraemia and hypernatraemia can be challenging. Multiple factors can cause results that are ‘fake’ or ‘spurious’ and not representative of the true in vivo sodium status. Additionally, although determining the volume status of patients with sodium homeostasis abnormalities is important for diagnosing the cause, this is often difficult to do in practice and can lead to misclassification if relied upon. Here, we present diagnostic algorithms that we hope will help the reader to systematically consider causes of sodium disturbances in a logical order. However, we appreciate that each patient case is different, and it may be appropriate to skip ahead to steps later in the algorithms, refer to other algorithms (e.g., potassium within this series), or even miss out steps entirely. These algorithms cannot replace specialist knowledge, experience and local guidelines. Instead, they should act as a diagnostic aid when assessing patients with sodium disturbances.
Acknowledgments
We would like to thank Professor Rousseau Gama for inspiring and inviting us to prepare this paper. All figures were created with BioRender.com.
Funding: None.
Footnote
Provenance and Peer Review: This article was commissioned by the editorial office, Journal of Laboratory and Precision Medicine for the series “Investigative Algorithms in Laboratory Medicine—Electrolytes and Acid/Base”. The article has undergone external peer review.
Reporting Checklist: The authors have completed the Narrative Review reporting checklist. Available at https://jlpm.amegroups.com/article/view/10.21037/jlpm-22-11/rc
Conflicts of Interest: All authors have completed the ICMJE uniform disclosure form (available at https://jlpm.amegroups.com/article/view/10.21037/jlpm-22-11/coif). The series “Investigative Algorithms in Laboratory Medicine—Electrolytes and Acid/Base” was commissioned by the editorial office without any funding or sponsorship. KS served as the unpaid Guest Editor of the series. The authors have no other conflicts of interest to declare.
Ethical Statement: The authors are accountable for all aspects of the work in ensuring that questions related to the accuracy or integrity of any part of the work are appropriately investigated and resolved.
Open Access Statement: This is an Open Access article distributed in accordance with the Creative Commons Attribution-NonCommercial-NoDerivs 4.0 International License (CC BY-NC-ND 4.0), which permits the non-commercial replication and distribution of the article with the strict proviso that no changes or edits are made and the original work is properly cited (including links to both the formal publication through the relevant DOI and the license). See: https://creativecommons.org/licenses/by-nc-nd/4.0/.
References
- Bie P. Mechanisms of sodium balance: total body sodium, surrogate variables, and renal sodium excretion. Am J Physiol Regul Integr Comp Physiol 2018;315:R945-62. [Crossref] [PubMed]
- Strazzullo P, Leclercq C. Sodium. Adv Nutr 2014;5:188-90. [Crossref] [PubMed]
- Frame AA, Wainford RD. Renal sodium handling and sodium sensitivity. Kidney Res Clin Pract 2017;36:117-31. [Crossref] [PubMed]
- Matsubara M. Renal sodium handling for body fluid maintenance and blood pressure regulation. Yakugaku Zasshi 2004;124:301-9. [Crossref] [PubMed]
- Spasovski G, Vanholder R, Allolio B, et al. Clinical practice guideline on diagnosis and treatment of hyponatraemia. Eur J Endocrinol 2014;170:G1-47. [Crossref] [PubMed]
- Hannon MJ, Verbalis JG. Sodium homeostasis and bone. Curr Opin Nephrol Hypertens 2014;23:370-6. [Crossref] [PubMed]
- Booth RE, Johnson JP, Stockand JD. Aldosterone. Adv Physiol Educ 2002;26:8-20. [Crossref] [PubMed]
- Fyhrquist F, Saijonmaa O. Renin-angiotensin system revisited. J Intern Med 2008;264:224-36. [Crossref] [PubMed]
- Nielsen S, DiGiovanni SR, Christensen EI, et al. Cellular and subcellular immunolocalization of vasopressin-regulated water channel in rat kidney. Proc Natl Acad Sci U S A 1993;90:11663-7. [Crossref] [PubMed]
- Williams DM, Gallagher M, Handley J, et al. The clinical management of hyponatraemia. Postgrad Med J 2016;92:407-11. [Crossref] [PubMed]
- Oster JR, Singer I. Hyponatremia, hyposmolality, and hypotonicity: tables and fables. Arch Intern Med 1999;159:333-6. [Crossref] [PubMed]
- Aw TC, Kiechle FL. Pseudohyponatremia. Am J Emerg Med 1985;3:236-9. [Crossref] [PubMed]
- El Hage L, Reineks E, Nasr C. Pseudohyponatremia in the setting of hypercholesterolemia. AACE Clin Case Rep 2018;5:e172-74. [Crossref] [PubMed]
- Ko GT, Yeung VT, Chow CC, et al. Pseudohyponatraemia secondary to hypercholesterolaemia. Ann Clin Biochem 1997;34:324-5. [Crossref] [PubMed]
- Fortgens P, Pillay TS. Pseudohyponatremia revisited: a modern-day pitfall. Arch Pathol Lab Med 2011;135:516-9. [Crossref] [PubMed]
- Nilsen J, Trabjerg E, Grevys A, et al. An intact C-terminal end of albumin is required for its long half-life in humans. Commun Biol 2020;3:181. [Crossref] [PubMed]
- Wilson DF, Matschinsky FM. Ethanol metabolism: The good, the bad, and the ugly. Med Hypotheses 2020; Epub ahead of print. [Crossref] [PubMed]
- Hillier TA, Abbott RD, Barrett EJ. Hyponatremia: evaluating the correction factor for hyperglycemia. Am J Med 1999;106:399-403. [Crossref] [PubMed]
- Purssell RA, Pudek M, Brubacher J, et al. Derivation and validation of a formula to calculate the contribution of ethanol to the osmolal gap. Ann Emerg Med 2001;38:653-9. [Crossref] [PubMed]
- Liamis G, Milionis H, Elisaf M. A review of drug-induced hyponatremia. Am J Kidney Dis 2008;52:144-53. [Crossref] [PubMed]
- Sanghvi SR, Kellerman PS, Nanovic L. Beer potomania: an unusual cause of hyponatremia at high risk of complications from rapid correction. Am J Kidney Dis 2007;50:673-80. [Crossref] [PubMed]
- Draper SB, Mori KJ, Lloyd-Owen S, et al. Overdrinking-induced hyponatraemia in the 2007 London Marathon. BMJ Case Rep 2009;2009:bcr09. [Crossref] [PubMed]
- Almond CS, Shin AY, Fortescue EB, et al. Hyponatremia among runners in the Boston Marathon. N Engl J Med 2005;352:1550-6. [Crossref] [PubMed]
- Hoorn EJ, Halperin ML, Zietse R. Diagnostic approach to a patient with hyponatraemia: traditional versus physiology-based options. QJM 2005;98:529-40. [Crossref] [PubMed]
- Kavouras SA. Assessing hydration status. Curr Opin Clin Nutr Metab Care 2002;5:519-24. [Crossref] [PubMed]
- Armstrong LE. Assessing hydration status: the elusive gold standard. J Am Coll Nutr 2007;26:575S-84S. [Crossref] [PubMed]
- Arasaradnam RP, Brown S, Forbes A, et al. Guidelines for the investigation of chronic diarrhoea in adults: British Society of Gastroenterology, 3rd edition. Gut 2018;67:1380-99.
- Chan S, Debono M. Replication of cortisol circadian rhythm: new advances in hydrocortisone replacement therapy. Ther Adv Endocrinol Metab 2010;1:129-38. [Crossref] [PubMed]
- Martin-Grace J, Dineen R, Sherlock M, et al. Adrenal insufficiency: Physiology, clinical presentation and diagnostic challenges. Clin Chim Acta 2020;505:78-91. [Crossref] [PubMed]
- Charmandari E, Nicolaides NC, Chrousos GP. Adrenal insufficiency. Lancet 2014;383:2152-67. [Crossref] [PubMed]
- El-Maouche D, Arlt W, Merke DP. Congenital adrenal hyperplasia. Lancet 2017;390:2194-210. [Crossref] [PubMed]
- Han TS, Walker BR, Arlt W, et al. Treatment and health outcomes in adults with congenital adrenal hyperplasia. Nat Rev Endocrinol 2014;10:115-24. [Crossref] [PubMed]
- Palmer BF. Cerebral salt wasting [Internet]. UpToDate. 2020. Available online: https://www.uptodate.com/contents/cerebral-salt-wasting?search=cerebral%20salt%20wasting&source=search_result&selectedTitle=1~21&usage_type=default&display_rank=1
- GRIBETZ I. RITTER S, GRAND MJ. Salt-losing nephritis. AMA J Dis Child 1958;96:191-6.
- Cuesta M, Thompson CJ. The syndrome of inappropriate antidiuresis (SIAD). Best Pract Res Clin Endocrinol Metab 2016;30:175-87. [Crossref] [PubMed]
- Yasir M, Mechanic OJ. Syndrome of Inappropriate Antidiuretic Hormone Secretion [Internet]. Treasure Island (FL): StatPearls Publishing, 2021.
- Kidney Disease: Improving Global Outcomes (KDIGO) Work Group. KDIGO Clincal Practice Guideline for Acute Kidney Injury. Kidney Int Suppl 2012;2:1-138.
- Kidney Disease: Improving Global Outcomes (KDIGO) Work Group. KDIGO 2012 Clinical Practice Guideline for the Evaluation and Management of Chronic Kidney Disease. Kidney Int Suppl 2013;3:1-150.
- John S, Thuluvath PJ. Hyponatremia in cirrhosis: pathophysiology and management. World J Gastroenterol 2015;21:3197-205. [Crossref] [PubMed]
- Vallet-Pichard A, Mallet V, Nalpas B, et al. FIB-4: an inexpensive and accurate marker of fibrosis in HCV infection. comparison with liver biopsy and fibrotest. Hepatology 2007;46:32-6. [Crossref] [PubMed]
- Lichtinghagen R, Pietsch D, Bantel H, et al. The Enhanced Liver Fibrosis (ELF) score: normal values, influence factors and proposed cut-off values. J Hepatol 2013;59:236-42. [Crossref] [PubMed]
- Adrogué HJ. Hyponatremia in Heart Failure. Methodist Debakey Cardiovasc J 2017;13:40. [Crossref] [PubMed]
- King M, Kingery J, Casey B. Diagnosis and evaluation of heart failure. Am Fam Physician 2012;85:1161-8. [PubMed]
- Hull RP, Goldsmith DJ. Nephrotic syndrome in adults. BMJ 2008;336:1185-9. [Crossref] [PubMed]
- DeFronzo RA. Hyperkalemia and hyporeninemic hypoaldosteronism. Kidney Int 1980;17:118-34. [Crossref] [PubMed]
- Talapatra I, Kalavalapalli S, Tymms DJ. Isolated hypoaldosteronism: An overlooked cause of hyponatraemia. Eur J Intern Med 2007;18:246-8. [Crossref] [PubMed]
- Solares I, Tejedor M, Jericó D, et al. Management of hyponatremia associated with acute porphyria-proposal for the use of tolvaptan. Ann Transl Med 2020;8:1098. [Crossref] [PubMed]
- Marsden JT, Rees DC. Urinary excretion of porphyrins, porphobilinogen and δ-aminolaevulinic acid following an attack of acute intermittent porphyria. J Clin Pathol 2014;67:60-5. [Crossref] [PubMed]
- Sahay M, Sahay R. Hyponatremia: A practical approach. Indian J Endocrinol Metab 2014;18:760-71. [Crossref] [PubMed]
- Adrogué HJ, Madias NE. Hypernatremia. N Engl J Med 2000;342:1493-9. [Crossref] [PubMed]
- Lang T, Prinsloo P, Broughton AF, et al. Effect of low protein concentration on serum sodium measurement: pseudohypernatraemia and pseudonormonatraemia! Ann Clin Biochem 2002;39:66-7. [Crossref] [PubMed]
- Chow E, Fox N, Gama R. Effect of low serum total protein on sodium and potassium measurement by ion-selective electrodes in critically ill patients. Br J Biomed Sci 2008;65:128-31. [Crossref] [PubMed]
- Goldwasser P, Ayoub I, Barth RH. Pseudohypernatremia and pseudohyponatremia: a linear correction. Nephrol Dial Transplant 2015;30:252-7. [Crossref] [PubMed]
- Dimeski G, Morgan TJ, Presneill JJ, et al. Disagreement between ion selective electrode direct and indirect sodium measurements: estimation of the problem in a tertiary referral hospital. J Crit Care 2012;27:326.e9-16. [Crossref] [PubMed]
- Lamb EJ, Abbas NA. Spurious hypernatraemia and Citra-Lock. Ann Clin Biochem 2007;44:579. [Crossref] [PubMed]
- Jeffery J, Ayling RM. A simple method for excluding possible Citra-lock contamination. Ann Clin Biochem 2008;45:448. [Crossref] [PubMed]
- Cornes MP, Ford C, Gama R. Undetected spurious hypernatraemia wastes health-care resources. Ann Clin Biochem 2011;48:87-8. [Crossref] [PubMed]
- Liamis G, Milionis HJ, Elisaf M. A review of drug-induced hypernatraemia. NDT Plus 2009;2:339-46. [PubMed]
- Christ-Crain M, Bichet DG, Fenske WK, et al. Diabetes insipidus. Nat Rev Dis Primers 2019;5:54. [Crossref] [PubMed]
- Di Iorgi N, Napoli F, Allegri AEM, et al. Diabetes insipidus - Diagnosis and management. Horm Res Paediatr 2012;77:69-84. [Crossref] [PubMed]
- Bolignano D, Cabassi A, Fiaccadori E, et al. Copeptin (CTproAVP), a new tool for understanding the role of vasopressin in pathophysiology. Clin Chem Lab Med 2014;52:1447-56. [Crossref] [PubMed]
- Lang F. Osmotic diuresis. Ren Physiol 1987;10:160-73. [PubMed]
- Lindner G, Schwarz C, Funk GC. Osmotic diuresis due to urea as the cause of hypernatraemia in critically ill patients. Nephrol Dial Transplant 2012;27:962-7. [Crossref] [PubMed]
Cite this article as: Flowers KC, Darragh-Hickey C, Kaur S, Allen GT, Shipman AR, Shipman KE. Investigative algorithms for disorders affecting plasma sodium: a narrative review. J Lab Precis Med 2022;7:26.