Investigative algorithms for disorders affecting human plasma alkaline phosphatase: a narrative review
Introduction
The alkaline phosphatases (ALPs) are a group of isoenzymes that catalyse the hydrolysis of organic phosphate esters. Located primarily in the cytoplasm they are anchored to the plasma membrane by a glycosylphosphatidylinositol anchor in almost all tissues (1,2), with a half-life of 7 days (3). Optimal enzyme conditions include an alkaline pH, between 8–11 (4). The ALP metalloenzyme family is encoded by multiple genes in humans, is expressed in multiple tissues including liver and bone, and each enzyme requires three metal ions, two Zn2+ and one Mg2+, in its active site (5).
ALP is widely included in panels designed to assess liver and bone function with alanine aminotransaminase (ALT) and/or aspartate aminotransferase (AST), total protein, albumin, and bilirubin, or calcium, albumin with or without phosphate respectively (6). The International Federation of Clinical Chemistry (IFCC) stated adult range is 45–135 U/L (7). Diagnostic algorithms will be presented to provide an approach to investigate abnormalities of ALP activity. This article is not meant to replace current guidelines and reviews, nor replace thorough clinical assessment. Instead, these algorithms are aimed to enhance the understanding of the role of diagnostics in the clinical pathway. Quality and efficiency of patient care are promoted by the appropriate use of diagnostics. We present this article in accordance with the Narrative Review reporting checklist (available at https://jlpm.amegroups.org/article/view/10.21037/jlpm-23-63/rc).
Methods
The narrative literature review was undertaken with review of PubMed, OMIM, ScienceDirect, and Google and seminal texts (Table 1). The search was performed from February 2023 to June 2023 from database inception. Language was restricted to English. Diagnostic algorithms were created from synthesis of the information obtained from literature review.
Table 1
Items | Specification |
---|---|
Date of search | February 2023–June 2023 |
Databases and other sources searched | PubMed, OMIM, ScienceDirect, Google |
Search terms used | “ALP”, “Alkaline Phosphatase”, “Hypophosphatasia”, “isoenzyme”, “pregnancy” |
Timeframe | From database inception to June 2023 |
Inclusion criteria | All papers and reviews were included, restricted to English |
Selection process | M.I. and K.E.S. conducted initial search, with refinement by all other authors to obtain consensus and agreement |
Any additional considerations, if applicable | Seminal texts were also searched and the references of important articles and texts were obtained and checked for relevance |
Metabolic role of ALP
ALP is a zinc metalloenzyme; it is activated by magnesium, or other cations. Encoded by four distinct genes that encode the isoforms found in humans with a great many functions (Table 2) (14). The most abundant, ALPL, accounts for the tissue non-specific ALP (TNSALP) that is found in liver, bone, and kidney, encoded on chromosome 1. Post translational modification further results in distinct carbohydrate compositions between those produced in the liver (hepatocellular and biliary canalicular subtypes) and in bone osteoblasts (BALP) (5). ALPP, ALPP2 and ALPI encode tissue specific ALP found in the placenta (syncitiotrophoblasts), germ cells, and intestines (enterocyte luminal surface) respectively (5). Tumours have been associated with excretion of variations of the placental isoform e.g., Regan isoenzyme (15). Both liver and intestinal types are found in the brush border of the renal proximal tubule (Table 2). The three major substrates for ALP are inorganic pyrophosphate (PPi), pyridoxal-5-phosphate (PLP), and phosphoethanolamine (PEA) (16).
Table 2
Human genes | Names | Tissue distribution | Function |
---|---|---|---|
ALPL | Tissue nonspecific alkaline phosphatase | Developing nervous system | Hydrolyses pyrophosphate supplying inorganic phosphate for mineralization, reduces extracellular pyrophosphate and phosphorylcholine concentration, dephosphorylation and detoxification of lipopolysaccharides, sphingosine 1-phosphate receptor signalling, antiendotoxin mediator and anti-inflammatory, regulation of adenosine concentrations |
Liver-bone-kidney type alkaline phosphatase | Skeletal tissue, liver, kidney | Hydrolyses a variable spectrum of phosphate-containing compounds, contributes to DNA synthesis, attenuates inflammation, influences mitochondrial respiration, extracellular matrix mineralization | |
ALPP | Placental alkaline phosphatase | Syncytio-trophoblast, tumours | Indicative of tissue having stem cell functions, tumour marker, detoxification of bacterial endotoxin |
ALPP2 | Germ cell alkaline phosphatase | Testis, malignant trophoblast, testicular cancer | Indicative of tissue having stem cell functions, sperm glycolytic reactions and fructose formation, tumour marker to diagnose carcinoma-in situ of the testis, seminoma |
ALPI | Intestinal alkaline phosphatase | Gut | Intestinal tight junction integrity and maintains barrier function, attenuates inflammation, regulation of intestinal surface pH, absorption of lipids, detoxification of free nucleotides and bacterial lipopolysaccharides, possible modulation of the gut microbiota, regulation of transmucosal passage of bacteria, dephosphorylation of extracellular adenosine triphosphate |
Although ubiquitous there is a considerable difference in relative enzyme activity between tissues (Table 3) (8). Although activity may be higher in the kidney, bone is heavier therefore the highest ALP tissue activity in total is found in the placenta followed by the intestine, bone, kidney, and liver in descending order (2). In health, human serum contains predominantly bone and liver isoforms, with approximately equal activity from both. Elevations of ALP activity are seen in health, e.g., during pregnancy and placenta formation (placental isoform), growing (bone isoform, and see later section on special states) or can represent a wide range of disorders of tissues (particularly those with high ALP activity) including endocrine and medication causes (2).
Table 3
Tissue | Activity per g of wet tissue with two substrates, liver activity set to 100 as benchmark | IU/g of tissue, mean ± standard deviation | |
---|---|---|---|
Β-glycerophosphate | Phenylphosphate | ||
Placenta | – | 3,214 | 69±44 |
Ileum (mucosa) | 1,714 | 2,524 | 38±14 |
Kidney | 619 | – | 2.1±0.7 |
Bone | – | 571 | Age dependent |
Colon (mucosa) | 471 | – | 2.3±0.8 |
Adrenal | 167 | – | |
Lung | 129 | – | 2.1±0.5 |
Spleen | 129 | – | |
Liver | 100 | 100 | 2.6±1.4 |
Brain | 76 | – | |
Stomach (mucosa) | 62 | – | |
Heart | – | 33 | |
Pancreas | – | 10 | |
Skeletal muscle | – | 5 | |
Serum (adult) | 0.2 | – | |
Testes | – | – | 0.5±0.1 |
Liver based ALP is synthesised in many tissues including hepatocytes and osteoblasts (Figures 1,2) (3,14). At least 90% (8) of the ALP is attached to exterior surfaces, 3% is found in the cytosol and the rest in the extracellular fluid and vessels. ALP is eliminated by being taken up by hepatocytes and catabolised in lysosomes (14) (Figure 1). In adults, there is a continuous process of bone remodelling, involving the resorption of bone by osteoclasts, followed by the synthesis and maintenance of bone by osteoblasts (Figure 2). This is a process that is regulated by complex interactions between large numbers of factors and hormones and is highly co-ordinated (18). ALP, no matter the source, is not cleared by the kidneys making it a useful tool for assessment of bone turnover in the presence of chronic kidney disease (CKD) (19).
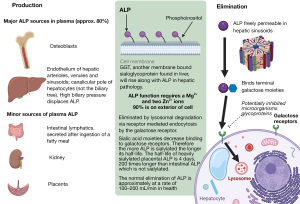
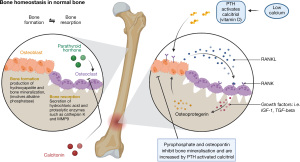
In CKD, abnormalities of mineral and bone metabolism occur with extra-skeletal calcification, called CKD-mineral bone disorder (CKD-MBD), which is distinct from the morphological diagnosis of renal osteodystrophy which is a consequence of it (20,21). Fibroblast growth factor 23 (FGF23) is raised prior to the more characteristic findings of hyperphosphataemia, high parathyroid hormone (PTH) or low activated vitamin D (1,25VitD) and is likely a key factor for renal, bone, mineral, and cardiovascular complications (22). Although FGF23 is phosphaturic, in CKD the renal response is diminished resulting in hyperphosphataemia which stimulates PTH secretion with a subsequent increase in bone turnover and measurable ALP activity. Furthermore, the impaired urinary tubular function and elevated serum FGF23 concentration lead to decreased vitamin D activation and enhanced PTH secretion under conditions of relative calcium deficiency, thereby giving rise to secondary hyperparathyroidism. BALP may be slightly superior in diagnosing bone disease in CKD-MBD but not enough to justify the additional cost for routine practice (21).
Measurement of ALP
ALP activity is dependent on zinc and activated by magnesium thereby order of blood draw is important to prevent cation chelation, and hence reduction in measured activity, by common tube additives, such as ethylenediaminetetraacetic acid (EDTA), oxalate, or citrate (23). Delayed separation of serum from the cells may very marginally increase ALP activity but analysis within 24 hours is unlikely to affect results in a clinically significant way irrespective if stored at room temperature or refrigerated (24,25).
The activity of ALP is measured by the change of absorbance at 405 nm by the yellow quinoid version of 4-nitrophenoxide formed at an alkaline pH following ALP catalysing the cleavage of phosphate from 4-nitrophenyl phosphate. The rate can be increased by including a phosphate acceptor e.g., adenosine monophosphate, which is also included in the IFCC reference method based on the above reaction (26).
Isoforms can be detected through a variety of means e.g., electrophoresis (27), differential deactivation e.g., by heat (1,28), differential response to inhibitors (1,29), affinity for lectins (30,31) and immunoassay (32,33). However, in clinical practice other routinely available tests are mostly reliable at identifying the source (as well as the marked improvement in imaging technology and availability) and the time, and cost, it may take to get these specialist tests makes them almost obsolete (34).
There are several caveats to consider when interpreting ALP results:
- Race and sex can impact ALP activity: positive correlation with increases in mean body mass index (BMI) of populations, seen in those of Hispanic descent greater than those of African American, which were greater than those of Caucasian. Males have higher activities than females within populations, which is also positively correlated to BMI (35).
- People who smoke have activity 10% higher than those who do not (36).
- Activity fluctuates approximately 6% from week to week in a healthy individual (37).
ALP can become bound to immunoglobulin, called macro-ALP, which prevents it being cleared as quickly (38,39). This is analytically correct, i.e., there is an increase in ALP activity in the serum, but does not represent increased tissue turnover and therefore a potential cause of spurious results.
Low ALP activity
In a study of unselected male patients, the causes of a low ALP, which was rare, occurring in only 0.2% of almost 70,000 samples, were:
- Cardiac surgery and cardiopulmonary bypass (26.5%), mean pre-surgical ALP was 71 U/L which fell to 20 U/L, corresponding magnesium concentration fell from a mean of 0.98 to 0.54 mmol/L.
- Malnutrition (12.0%), mean ALP of 18 U/L, secondary to decreased activity of both bone and hepatic ALP.
- Severe magnesium deficiency (mean concentration 0.48 mmol/L) affected 4.8% with a mean ALP activity of 21 U/L.
- Hypothyroidism (2.4%), ALP activity returning to normal once euthyroid.
- Severe anaemia attributed to iron deficiency (1.2%) (40).
In an audit of a year’s cases another group identified blood transfusion, cardiopulmonary bypass, and chemotherapy as causes, but a few cases had no identifiable cause (41). This led the team to conclude that the lower limit of ALP activity is too arbitrary to be useful to pick up important pathology. The causes of low ALP activity will be discussed below with Figure 3 providing a diagnostic algorithm aimed at helping the clinician approach the investigation.
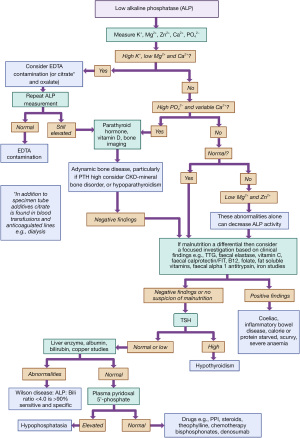
Pseudo hypophosphatasia
For an unexpected isolated low ALP activity EDTA, or other tube additives such as oxalate or citrate, contamination should be considered. Serum ALP activity decreased significantly at EDTA concentrations of >1.86 mmol/L (unexpected hyperkalaemia and hypocalcaemia are further clues) but this degree of contamination is not commonly reached in most cases of contamination (42,43). EDTA, citrate and oxalate bind cations and hence reduce ALP activity which requires both zinc and magnesium ions. Citrate is also found in blood transfusions and anticoagulated lines, e.g., in haemodialysis.
Primary hypophosphatasia
Primary low ALP activity, called hypophosphatasia, is due to genetic mutations in the ALPL gene (TNSALP) and clinical symptoms can include rickets and osteomalacia, epilepsy, myopathy, respiratory difficulties, hypercalcaemia, nephrocalcinosis, and tooth loss (16). At its most severe it can be fatal in infancy, but others will present as adults (16). Despite the wide phenotype all will have dental and skeletal mineralization symptoms, with high PPi in the bone matrix (16). Deformed and painful bones in infancy or painless, early, tooth loss (with roots attached) may indicate hypophosphatasia (16). ALP deactivates active B6, PLP, to form pyridoxal, and the accumulation of pyridoxal may account for the seizures seen in some babies with hypophosphatasia, occurring a few days after birth. Although not demonstrated in humans, restoring ALP activity in deficient mice resolves the seizures (16). High urinary PEA is another useful screening tool however genetic diagnostics (more than 300 mutations have been described in the ALPL gene but not all are disease causing) and enzyme replacement therapy is now available (16). Hyperphosphataemia can be seen, and later hypercalcaemia, as the phosphate and calcium homeostasis mechanisms are normal promoting renal excretion which can lead to renal complications such as renal failure and nephrocalcinosis (16).
Malnutrition and malabsorption
Malnutrition can lead to low ALP activity. This may be caused by metal deficiencies such as zinc or magnesium (44,45). In one study all those with a low ALP, compared to controls, were deficient in either zinc (47.6%) or magnesium (52.4%) (46). Nutritional rickets can reduce ALP activity, however if vitamin D deficient with low dietary calcium intake (47), ALP may be elevated (although not reliably) (48-50). A low protein state may lead to a low ALP activity (51,52) but again this observation is not consistent in kwashiorkor (53).
Vitamins C and B12 have been shown to promote bone growth, and deficiencies have a negative impact on bone growth (54) and increase the rate of osteoporosis (55). ALP is a marker for bone turnover therefore any decrease in turnover will affect the activity of ALP (55). However, it is unusual to find isolated nutritional deficiencies with, for example, a case report of scurvy demonstrating elevated ALP due to hypovitaminosis D, and coeliac disease which is often associated with elevated ALP activity (56,57).
Endocrine and metabolic links to ALP
Wilson disease, an inborn error of copper metabolism, has been associated with low ALP activity, particularly in the initial stages (58). It is believed that copper competition with zinc causes the suppression in ALP activity (25). Conditions associated with low bone turnover, such as hypoparathyroidism and hypothyroidism, may reduce ALP activity, likely not enough to cause low ALP activity commonly (16,59). In cardiopulmonary bypass ALP is presumed to be consumed by dephosphorylating inflammatory chemicals and the reduction in activity correlates with worsening outcomes (60).
Drugs and toxins
Clofibrate reduces ALP activity but is no longer used (61,62), having been replaced by other drugs in the fibrate class due to side effects. ALP activity is inhibited by theophylline, sulphonamides, arsenates, molybdates, and other agents, with active development of ALP inhibitors underway to attempt to treat ectopic calcification which are not yet on the market (8,63-65). Inhibition by cation chelation by citrate can cause a transiently low ALP, e.g., in neonates or massive transfusions (41). ALP production is reduced by bisphosphonates (66,67), denosumab (68), and proton pump inhibitors (69) although they may not result in ALP activity beneath the reference range (70) (see Table 4).
Table 4
Drugs | Mechanism |
---|---|
Proton pump inhibitors | Inhibit osteoblasts, decreases bone turnover |
Steroids | Effects variable on bone turnover and can also raise ALP |
Theophylline, aminophylline | Inhibits ALP activity and so test is falsely low |
Bisphosphonates | Suppress osteoclast and so negative feedback to osteoblast |
Denosumab | Suppress osteoclast and so negative feedback to osteoblast |
Sulfonamides | Inhibits ALP activity and so test is falsely low |
Cimetidine and ranitidine | Inhibits ALP activity and so test is falsely low |
Imidazole and levamizole | Inhibits ALP activity and so test is falsely low |
Nitrofurantoin, cyanides, arsenals | Inhibits ALP activity and so test is falsely low |
ALP, alkaline phosphatase.
High ALP
The commonest tissue origins of an elevated ALP activity are liver and bone (see Table 5). The key differentials will differ depending on how well the person is and, potentially, the degree of elevation (Table 5). In hospitalised patients ALP is commonly raised [causes include pyelonephritis, malignancy, congestive heart failure and renal failure (14,78,79)]; in those with underlying conditions (who are well) elevations tend to resolve within 3 months (14). The causes of a rise in ALP activity are discussed below. A diagnostic algorithm is provided as a systematic framework that can be used to guide rather than be followed proscriptively, see Figure 4 (80,81). It is important to note in mild elevations of ALP activity other laboratory tests may not be helpful in identifying the cause, particularly in asymptomatic patients, and there may need to be a strategy of watchful waiting to determine if the elevation is persistent and the organ source [if less than 1.5 times the upper limit of normal (ULN) for example consider repeating at 3 months if isolated and patient is well] (82).
Table 5
Disorder | Patients with abnormal ALP (%) | Mean ALP (multiple of upper reference limit) |
---|---|---|
Primary liver cancer | 92 | 5.5 |
Tumour, metastatic liver | 88 | 5.5 |
Extrahepatic obstruction | 94 | 4.9 |
Intrahepatic obstruction | 82 | 2.8 |
Acute viral hepatitis | 80 | 2.5 |
Inactive cirrhosis | 75 | 2.1 |
Alcoholic hepatitis | 77 | 1.8 |
Chronic active hepatitis | 78 | 1.7 |
Primary biliary cholangitis | >95 | 1.67† |
Osseous metastases | 74 | 2 |
Pregnancy | 100 | Depends on stage, up to 4 |
Osteomalacia | 80 | 1.5 |
Low vitamin D | 0 | – |
Hyperthyroidism | 44 | Up to 5 |
Secondary hyperparathyroidism | 75 | – |
†, wide range of values, this figure, and above, is the trigger for treatment with ursodeoxycholic acid. ALP, alkaline phosphatase.
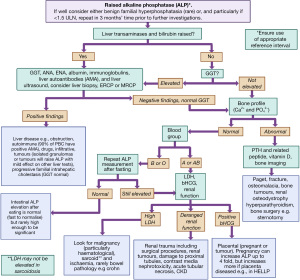
ALP and its association with the liver and biliary tract
Most test panels for liver function include the enzymes ALT and ALP. A larger rise in ALT activity (compared with the ULN) may indicate hepatocellular damage whereas a relatively more significant ALP elevation (compared with the ULN) likely indicates a cholestatic pathology. Gamma-glutamyl transferase (GGT) elevation supports the diagnosis of a liver aetiology (GGT should be normal in bone causes of high ALP, unless there is more than one pathology) (83-85). An exception is the rare familial intrahepatic cholestasis which has raised ALP and normal GGT activities (86). This can present in a benign form that occurs at any age, and lasts for several weeks to months, or a progressive form that causes severe cholestasis before 6 months of age and progressing to cirrhosis, liver failure and death, unless a liver transplant is provided (86).
When reviewing raised ALP results, an elevation of ALP of approximately four times the ULN or greater occurs in up to 75% of the patients with cholestasis, either intrahepatic or extrahepatic in one study (87). Liver diseases that principally effect parenchymal cells, such as infectious hepatitis, typically show only moderately elevated or even normal ALP activity but this depends upon study (see Table 5). As per the European Association for the Study of the Liver (EASL), activity thresholds for serum requiring diagnostic work-up are >1.5 times ULN (88).
This review will concentrate on the cholestatic liver diseases as the hepatocellular diseases will be covered in more detail in the companion article to this (on investigation of elevated transaminases). Cholestatic liver diseases include autoimmune e.g., primary biliary cholangitis or cirrhosis (PBC) (89). PBC is diagnosed by the presence of anti-mitochondrial antibodies (AMAs) (90) and increased concentrations of immunoglobulins [mainly immunoglobulin M (IgM)] (91). Anti-nuclear antibodies (ANAs) and anti-smooth muscle antibodies (SMAs) are found in nearly 50% of PBC patients (92). ANA, anti-glycoprotein 210 and/or anti-sp100 (nuclear membrane proteins) may be present in those who are AMAs negative (93).
Primary sclerosing cholangitis (PSC) and secondary sclerosing cholangitis are differentials for PBC (94,95). Up to 80% of people with PSC are diagnosed with inflammatory bowel disease (IBD), primarily ulcerative colitis (88). In PSC immunoglobulins are often elevated with IgM (96) and IgG raised in 45% and 61% respectively, with IgG typically exceeding 1.5 times the ULN (97). Immunoglobulin G Subclass 4 (IgG4) was found to be elevated in 9% of PSC patients in another study and has been suggested to be a disease subtype (98,99), with those with raised IgG4 tending to progress more rapidly without treatment (98,100). Other autoantibodies present include perinuclear antineutrophil cytoplasmic antibodies (pANCAs) (26–94%), ANA (8–77%), and SMAs (0–83%) (101).
Imaging is relevant for all patients in whom cholestasis is suspected, cholangiography, preferentially with non-invasive magnetic resonance imaging (MRI), or endoscopically, to exclude PSC. Transient elastography is another non-invasive tool that has shown high degree of accuracy in diagnosing advanced fibrosis in patients with PBC (102). A liver biopsy is rarely required.
Obstruction of the biliary tree, or cholestasis, will lead to an elevation of the ALP and causes for this include gallstone, biliary strictures and tumours, infiltrative processes, and medication (see Table 6) (82,108-111). For example, colestipol, a bile acid sequestrant, increases ALP activity but is not widely used anymore (61).
Table 6
Drugs | Mechanism |
---|---|
Antibiotics | |
Penicillin derivatives | Intrahepatic cholestasis |
Erythromycin | Intrahepatic cholestasis |
Aminoglycosides | Enzyme induction |
Sulfa drugs | Intrahepatic cholestasis |
Antiepileptic drugs | |
Carbamazepine | Intrahepatic cholestasis |
Phenobarbital | Enzyme induction |
Phenytoin | Enzyme induction |
Sodium valproate | Enzyme induction |
Antihistamines | |
Cetirizine | Intrahepatic cholestasis |
Cardiovascular drugs | |
Captopril | Intrahepatic cholestasis |
Diltiazem | Enzyme induction |
Felodipine | Enzyme induction |
Verapamil | Intrahepatic cholestasis |
Disease modifying agents | |
Penicillamine | Intrahepatic cholestasis |
Sulfa drugs | Intrahepatic cholestasis |
Allopurinol | Causes a granulomatous hepatitis |
Polycyclic aromatic hydrocarbons | |
Oral contraceptive pill (oestrogen) | Enzyme induction |
Anabolic and corticosteroids | Enzyme induction but variable and can lead to low ALP |
Psychotropic drugs | |
Monoamine oxidase inhibitors | Intrahepatic cholestasis |
Phenothiazines and chlorpromazine | Intrahepatic cholestasis |
Lipid lowering | |
Statins | Enzyme induction |
ALP, alkaline phosphatase.
Bone
Increasing bone turnover, particularly osteoblast activity, will elevate ALP (5). Other tests including vitamin D, calcium, phosphate and PTH may be required to distinguish some of the following disease entities, and this is further illustrated in Figure 4. There are other, less commonly requested tests, which might also be available for the clinician to distinguish bone diseases (see sister algorithms in this special series on calcium and phosphate).
Common causes of bone pathology that increases ALP are periods of increased skeletal growth, such as during adolescence (4), fracture recovery (112), cancer including osteosarcoma or bone metastasis, hyperparathyroidism, CKD and vascular calcification (113).
Rickets, a rare condition in the UK, is the clinical consequence of impaired mineralization of the growth plate cartilage and spongiosa in the metaphysis of children and adolescents (114) due to calcium and/or vitamin D deficiency (calcipenic rickets), renal tubule dysfunction and disturbances of chondrocytes and osteoblasts (Table 7). Bones are resorbed to release calcium, under PTH action, which also results in hypophosphataemia (116,117). Osteomalacia is rickets occurring after the growth plates fuse, i.e., the adult version (114), and biochemically there will be raised PTH with deficiencies in phosphate and vitamin D. In osteomalacia vitamin D supplementation will return ALP activity to normal after about 6 months of treatment, therefore there is no need to remeasure activity prior to this time point (34).
Table 7
Cause | Calcium | Vitamin D | PTH | Phosphate | Other |
---|---|---|---|---|---|
Hypovitaminosis D | L | L | H | L | H ALP |
Low 1,25D (renal failure, vitamin D dependent rickets type I caused by loss of function in 1α hydroxylase) | L | N | H | L/H | H ALP, L 1,25D |
Vitamin D dependent rickets type II (loss of function of vitamin D receptor) | L | N | H | L | H ALP, H 1,25D |
Low phosphate (multiple causes see later slide) | N | N | N | L | H ALP |
PTH, parathyroid hormone; L, low; H, high; N, normal; ALP, alkaline phosphatase.
Paget disease is an increasingly rare condition of disordered bone turnover with no clear single cause (118,119). Markers of bone turnover are raised including type I procollagen N-terminal peptide (P1NP), N-terminal telopeptide of type I collagen and bone ALP (120), with ALP activity used to measure treatment efficacy (121). However, there is an argument that ALP normalization is an inappropriate measure of treatment success as only occurred in ~25% of those treated with bisphosphonates, though ALP activity overall did reduce by ~41% (122). Treating to normalise ALP has no reported differences in fracture rate, pain relief, hearing, need for orthopaedic surgery or quality of life when compared to treating to alleviate bone pain solely (123,124). Imaging is used to confirm a diagnosis of Paget disease (125).
Osteoporosis is not associated with an elevation of ALP, unless there is a secondary fracture, however BALP can be used to monitor the effect of treatment on bone turnover (126). Osteomyelitis does not raise ALP either. A femoral neck fracture elevates serum ALP activity by approximately 30% and trochanteric by 100% (14,127).
Note that oestrogens reduce BALP in post-menopausal women which might theoretically disguise subtle elevations in women on hormone replacement therapy (128). Steroids have an unpredictable effect on BALP as although they may be osteogenic in exogenous or endogenous hypercortisolism there may be no noticeable effects or mild suppression (66,129,130). Hyperthyroidism and thyrotoxicosis cause an elevation in ALP, likely by effects on bone metabolism (131,132).
Tumour placental ALP
In cancer there can be significant production of ALP which cannot be related to the tissue involvement and therefore represents paraneoplastic production of foetal proteins (particularly placental type ALP) from the tumour. Regan isoform, a rare variant of placental ALP, is one example; in one series (133) of 239 people with malignant disease 25.5% had elevated Regan isoenzyme detectable in the serum. Tumour types reported to demonstrate elevations of Regan isoform include kidney, stomach, uterine, lung, cervical, endometrial, testicular, ovarian, medullary thyroid, haematopoietic, prostate and germ cell (15). Other examples include Kasahara a foetal intestinal ALP isoform (134) e.g., in renal cell carcinoma (135), and Nagao a placental-like ALP isoform (134,136).
Miscellaneous
Transient hyperphosphatasaemia (TH) is a benign condition, of unknown aetiology, characterized by marked elevation of serum ALP activity, such as an increase of four-fold ULN. There should be a notable absence of associated diseases such as liver, bone, or kidney pathology and it settles within weeks or months of initial observation (137). However, ALP activity can remain elevated for extended periods of time, for greater than 4 months, in approximately 20% of cases (138). Diagnosis of TH is linked to the ‘fast’ α2 band which is detected on agarose gel electrophoresis (139) but testing may not be necessary if the person is asymptomatic. Prevalence of TH is suggested to be around 2.8% (ALP >1,000 U/L) (140) in the classically affected population, children younger than 5 years old, but has also been described in transplant patients (141,142).
Bacterial and fungal glycoproteins can compete with the receptors involved in the excretion and elimination of ALP from the serum (14). Therefore, ALP elevations can be seen in infections without any obvious liver toxicity or cholestasis (143).
Raised ALP has also been identified in a range of other disorders including rheumatoid arthritis (144,145), atherosclerosis (146), and axial spondylarthritis (147). However, the links between elevated ALP and the diseases have not been fully established, or the mechanisms understood.
In individuals with blood groups O and B, ALP concentration increases by about 20% after consuming a fatty meal, due to contribution from the intestinal tract isoenzyme (14,148). It was found that red cells of blood group A bind almost all intestinal ALP (149), which is not replicated in those with types O or B. As this elevation can persist for up to 14 hours in the serum, the recommendation is to check the serum enzyme activity in a fasting state (150). In one case a patient’s intestinal ALP activity was measured as high as 140 U/L, with a normal range suggested to be <18 U/L (151). There are also cases of benign familial conditions causing elevated intestinal ALP. Rosalki et al. presents examples of patients with persistent and unexplained raised ALP, above the reference range, that had a genetic component, with an autosomal dominant pattern of inheritance suggested as the cause (152).
Special states
Serum ALP varies with age and gender. There are two peaks of ALP activity during infancy and puberty, which fall mid-childhood and towards the end of adolescence respectively (153,154). A German study of over 300,000 paediatric plasma samples demonstrated that at birth ALP activity is low. Activity increases quickly, peaking at 20 days and then decreasing again until 4 years of age with an increase during adolescence to reach adult ranges (155). For a reference source for paediatric values the CALIPER database is a useful source (156). Gender will also influence these peaks, with females shown to peak 2 years earlier than males in keeping with growth rates (112,138).
Gender also influences expected BALP activity in later life, significantly higher activities were found in postmenopausal women when compared to premenopausal (157). During pregnancy, bone turnover increases (158) contributing to an increase in BALP plus an additional increase in placental ALP activity in serum resulting in an ALP activity threefold higher at the end of term (159). Therefore, ALP activity should be reviewed against appropriate reference ranges to avoid over diagnosing or missing pathology.
Conclusions
Serum ALP activity can be affected by normal physiological states and diseases. Pathological causes of high activity commonly are attributable to bone or liver disorders. Unexpectedly low activity may be due to pre-analytical contamination with cation chelators. A set of diagnostic algorithms have been created to guide the reader’s approach and provide a systematic route of testing. Each patient is unique however and the clinical picture may direct the reader to skip steps or refer to other algorithms within the series or in the literature. These algorithms are not a replacement for experience, expert opinion or local guidelines and should instead act as a diagnostic aid.
Acknowledgments
All figures were created with BioRender.com.
Funding: None.
Footnote
Provenance and Peer Review: This article was commissioned by the editorial office, Journal of Laboratory and Precision Medicine for the series “Investigative Algorithms in Laboratory Medicine II: Focus on Bone and Liver”. The article has undergone external peer review.
Reporting Checklist: The authors have completed the Narrative Review reporting checklist. Available at https://jlpm.amegroups.org/article/view/10.21037/jlpm-23-63/rc
Peer Review File: Available at https://jlpm.amegroups.org/article/view/10.21037/jlpm-23-63/prf
Conflicts of Interest: All authors have completed the ICMJE uniform disclosure form (available at https://jlpm.amegroups.org/article/view/10.21037/jlpm-23-63/coif). The series “Investigative Algorithms in Laboratory Medicine II: Focus on Bone and Liver” was commissioned by the editorial office without any funding or sponsorship. K.E.S. served as the unpaid Guest Editor of the series and serves as an unpaid editorial board member of the Journal of Laboratory and Precision Medicine from September 2022 to August 2024. The authors have no other conflicts of interest to declare.
Ethical Statement: The authors are accountable for all aspects of the work in ensuring that questions related to the accuracy or integrity of any part of the work are appropriately investigated and resolved.
Open Access Statement: This is an Open Access article distributed in accordance with the Creative Commons Attribution-NonCommercial-NoDerivs 4.0 International License (CC BY-NC-ND 4.0), which permits the non-commercial replication and distribution of the article with the strict proviso that no changes or edits are made and the original work is properly cited (including links to both the formal publication through the relevant DOI and the license). See: https://creativecommons.org/licenses/by-nc-nd/4.0/.
References
- Sharma U, Pal D, Prasad R. Alkaline phosphatase: an overview. Indian J Clin Biochem 2014;29:269-78. [Crossref] [PubMed]
- Lott JA, Wolf PL, Nemesánszky E, et al. Clinical enzymology: a case-oriented approach. 1986:285.
- Lowe D, Sanvictores T, Zubair M, et al. Alkaline Phosphatase. Treasure Island, FL, USA: StatPearls Publishing; 2023.
- Golub EE, Boesze-Battaglia K. The role of alkaline phosphatase in mineralization. Curr Opin Orthop 2007;18:444-8. [Crossref]
- Makris K, Mousa C, Cavalier E. Alkaline Phosphatases: Biochemistry, Functions, and Measurement. Calcif Tissue Int 2023;112:233-42. [Crossref] [PubMed]
- Siller AF, Whyte MP. Alkaline Phosphatase: Discovery and Naming of Our Favorite Enzyme. J Bone Miner Res 2018;33:362-4. [Crossref] [PubMed]
- International Confederation of Clinical Chemistry. [cited 2023 Nov 7]. Available online: https://grid.ifcc.org/
- Le-Vinh B, Akkuş-Dağdeviren ZB, Le NMN, et al. Alkaline Phosphatase: A Reliable Endogenous Partner for Drug Delivery and Diagnostics. Advanced Therapeutics 2022;5:2100219. [Crossref]
- Millán JL. Alkaline Phosphatases: Structure, substrate specificity and functional relatedness to other members of a large superfamily of enzymes. Purinergic Signal 2006;2:335-41. [Crossref] [PubMed]
- Vimalraj S. Alkaline phosphatase: Structure, expression and its function in bone mineralization. Gene 2020;754:144855. [Crossref] [PubMed]
- Lallès JP. Recent advances in intestinal alkaline phosphatase, inflammation, and nutrition. Nutr Rev 2019;77:710-24. [Crossref] [PubMed]
- Zaher DM, El-Gamal MI, Omar HA, et al. Recent advances with alkaline phosphatase isoenzymes and their inhibitors. Arch Pharm (Weinheim) 2020;353:e2000011. [Crossref] [PubMed]
- Zhang Z, Nam HK, Crouch S, et al. Tissue Nonspecific Alkaline Phosphatase Function in Bone and Muscle Progenitor Cells: Control of Mitochondrial Respiration and ATP Production. Int J Mol Sci 2021;22:1140. [Crossref] [PubMed]
- Levitt MD, Hapak SM, Levitt DG. Alkaline Phosphatase Pathophysiology with Emphasis on the Seldom-Discussed Role of Defective Elimination in Unexplained Elevations of Serum ALP - A Case Report and Literature Review. Clin Exp Gastroenterol 2022;15:41-9. [Crossref] [PubMed]
- Bukowczan J, Pattman S, Jenkinson F, et al. Regan isoenzyme of alkaline phosphatase as a tumour marker for renal cell carcinoma. Ann Clin Biochem 2014;51:611-4. [Crossref] [PubMed]
- Linglart A, Biosse-Duplan M. Hypophosphatasia. Curr Osteoporos Rep 2016;14:95-105. [Crossref] [PubMed]
- McComb RB, Bowers GN, Posen S. Alkaline Phosphatase. Boston, MA, USA: Springer US; 1979.
- Burch J, Rice S, Yang H, et al. Systematic review of the use of bone turnover markers for monitoring the response to osteoporosis treatment: the secondary prevention of fractures, and primary prevention of fractures in high-risk groups. Health Technol Assess 2014;18:1-180. [Crossref] [PubMed]
- Sprague SM, Bellorin-Font E, Jorgetti V, et al. Diagnostic Accuracy of Bone Turnover Markers and Bone Histology in Patients With CKD Treated by Dialysis. Am J Kidney Dis 2016;67:559-66. [Crossref] [PubMed]
- Lewis R. Mineral and bone disorders in chronic kidney disease: new insights into mechanism and management. Ann Clin Biochem 2012;49:432-40. [Crossref] [PubMed]
- Moe S, Drüeke T, Cunningham J, et al. Definition, evaluation, and classification of renal osteodystrophy: a position statement from Kidney Disease: Improving Global Outcomes (KDIGO). Kidney Int 2006;69:1945-53. [Crossref] [PubMed]
- Vervloet MG. Shedding Light on the Complex Regulation of FGF23. Metabolites 2022;12:401. [Crossref] [PubMed]
- Banfi G, Salvagno GL, Lippi G. The role of ethylenediamine tetraacetic acid (EDTA) as in vitro anticoagulant for diagnostic purposes. Clin Chem Lab Med 2007;45:565-76. [Crossref] [PubMed]
- Selvakumar C, Madhubala V. Effect of sample storage and time delay (delayed processing) on analysis of common clinical biochemical parameters. Int J Clin Biochem Res 2017;4:295-8.
- Marshall W. Alkaline phosphatase (serum, plasma). Analyte Monograph. 2013.
- Schumann G, Klauke R, Canalias F, et al. IFCC primary reference procedures for the measurement of catalytic activity concentrations of enzymes at 37 °C. Part 9: reference procedure for the measurement of catalytic concentration of alkaline phosphatase International Federation of Clinical Chemistry and Laboratory Medicine (IFCC) Scientific Division, Committee on Reference Systems of Enzymes (C-RSE) (1)). Clin Chem Lab Med 2011;49:1439-46. [Crossref] [PubMed]
- Epstein E, Kiechle FL, Zak B. Use of alkaline phosphatase isoenzyme analysis in the evaluation of cholestatic liver disease. Ann Clin Lab Sci 1984;14:292-7. [PubMed]
- Moss DW, Whitby LG. A simplified heat-inactivation method for investigating alkaline phosphatase isoenzymes in serum. Clin Chim Acta 1975;61:63-71. [Crossref] [PubMed]
- Mulivor RA, Plotkin LI, Harris H. Differential inhibition of the products of the human alkaline phosphatase loci. Ann Hum Genet 1978;42:1-13. [Crossref] [PubMed]
- Anderson DJ, Branum EL, O'Brien JF. Liver- and bone-derived isoenzymes of alkaline phosphatase in serum as determined by high-performance affinity chromatography. Clin Chem 1990;36:240-6. [Crossref] [PubMed]
- Le Bricon T, Gay-Bellile C, Cottu P, et al. Lectin affinity electrophoresis of serum alkaline phosphatase in metastasized breast cancer. J Clin Lab Anal 2010;24:20-4. [Crossref] [PubMed]
- Hata K, Tokuhiro H, Nakatsuka K, et al. Measurement of bone-specific alkaline phosphatase by an immunoselective enzyme assay method. Ann Clin Biochem 1996;33:127-31. [Crossref] [PubMed]
- Broyles DL, Nielsen RG, Bussett EM, et al. Analytical and clinical performance characteristics of Tandem-MP Ostase, a new immunoassay for serum bone alkaline phosphatase. Clin Chem 1998;44:2139-47. [Crossref] [PubMed]
- Haji SM, Chipchase A, Fraser WD, et al. Retrospective evaluation of a local protocol used to enhance laboratory savings through minimizing the performance of alkaline phosphatase isoenzyme analysis. Ann Clin Biochem 2019;56:298-301. [Crossref] [PubMed]
- Gonzalez H, Imam Z, Wong R, et al. Normal alkaline phosphatase levels are dependent on race/ethnicity: NationalGEP Health and Nutrition Examination Survey data. BMJ Open Gastroenterol 2020;7:e000502. [Crossref] [PubMed]
- Wannamethee SG, Shaper AG. Cigarette smoking and serum liver enzymes: the role of alcohol and inflammation. Ann Clin Biochem 2010;47:321-6. [Crossref] [PubMed]
- EFLM Biological Variation. ALP Liver. [cited 2023 May 12]. Available online: https://biologicalvariation.eu/search?query=Alkaline%20phosphatase%20(ALP),%20liver%20type
- McTaggart MP, Rawson C, Lawrence D, et al. Identification of a macro-alkaline phosphatase complex in a patient with inflammatory bowel disease. Ann Clin Biochem 2012;49:405-7. [Crossref] [PubMed]
- Ramasamy I. Persistent Increase in Serum Alkaline Phosphatase in a Patient with Monoclonal Gammopathy of Undefined Significance. Case Rep Hematol 2020;2020:8406971. [Crossref] [PubMed]
- Lum G. Significance of low serum alkaline phosphatase activity in a predominantly adult male population. Clin Chem 1995;41:515-8. [Crossref] [PubMed]
- Macfarlane JD, Souverijn JH, Breedveld FC. Clinical significance of a low serum alkaline phosphatase. Neth J Med 1992;40:9-14. [PubMed]
- Kalaria T, Ford C, Gama R. Managing ethylenediaminetetraacetic acid (EDTA) interference in EDTA contaminated samples - selectivity in reporting analytes. Ann Clin Biochem 2023;60:92-9. [Crossref] [PubMed]
- White G. Serum ethylenediaminetetraacetic acid concentrations in routine samples submitted for biochemical analysis. Ann Clin Biochem 2010;47:485-6. [Crossref] [PubMed]
- Jain A, Jadhav AA, Varma M. Relation of oxidative stress, zinc and alkaline phosphatase in protein energy malnutrition. Arch Physiol Biochem 2013;119:15-21. [Crossref] [PubMed]
- Atinmo T, Johnson A, Mbofung C, et al. Plasma zinc status of protein energy malnourished children. Acta Trop 1982;39:265-74. [PubMed]
- Ray CS, Singh B, Jena I, et al. Low Alkaline Phosphatase (ALP) In Adult Population an Indicator of Zinc (Zn) and Magnesium (Mg) Deficiency. Curr Res Nutr Food Sci Jour 2017;5:347-52. [Crossref]
- Pettifor JM. Screening for nutritional rickets in a community. J Steroid Biochem Mol Biol 2016;164:139-44. [Crossref] [PubMed]
- Vasudevan J, Jenifer A, Reddy GM, et al. Serum alkaline phosphatase for screening of hypovitaminosis D. Indian Pediatr 2014;51:60-1. [PubMed]
- Taylor JA, Richter M, Done S, et al. The utility of alkaline phosphatase measurement as a screening test for rickets in breast-fed infants and toddlers: a study from the puget sound pediatric research network. Clin Pediatr (Phila) 2010;49:1103-10. [Crossref] [PubMed]
- Haffner D, Leifheit-Nestler M, Grund A, et al. Rickets guidance: part I-diagnostic workup. Pediatr Nephrol 2022;37:2013-36. [Crossref] [PubMed]
- Waterlow JC. Enzyme changes in malnutrition. J Clin Pathol Suppl (Assoc Clin Pathol) 1970;4:75-9. [Crossref] [PubMed]
- Kumari R, Rao YN, Talukdar B, et al. Serum enzyme abnormalities in protein energy malnutrition. Indian Pediatr 1993;30:469-73. [PubMed]
- Adejuwon CA, Akinyinka OO, Ayo-ola BM. Apparent hypocalcaemia in Nigerian children with kwashiorkor. West Afr J Med 1994;13:168-70. [PubMed]
- Choi HK, Kim GJ, Yoo HS, et al. Vitamin C Activates Osteoblastogenesis and Inhibits Osteoclastogenesis via Wnt/β-Catenin/ATF4 Signaling Pathways. Nutrients 2019;11:506. [Crossref] [PubMed]
- Kim GS, Kim CH, Park JY, et al. Effects of vitamin B12 on cell proliferation and cellular alkaline phosphatase activity in human bone marrow stromal osteoprogenitor cells and UMR106 osteoblastic cells. Metabolism 1996;45:1443-6. [Crossref] [PubMed]
- Fehlmann HU. Scurvy in an adult. Schweiz Med Wochenschr 1977;107:1199-202. [PubMed]
- Villavicencio Kim J, Wu GY. Celiac Disease and Elevated Liver Enzymes: A Review. J Clin Transl Hepatol 2021;9:116-24. [PubMed]
- Shaver WA, Bhatt H, Combes B. Low serum alkaline phosphatase activity in Wilson's disease. Hepatology 1986;6:859-63. [Crossref] [PubMed]
- Weber G, Mora S, Bellini A, et al. Bone mineral metabolism and thyroid replacement therapy in congenital hypothyroid infants and young children. J Endocrinol Invest 1995;18:277-82. [Crossref] [PubMed]
- Schaefer AK, Hutschala D, Andreas M, et al. Decrease in serum alkaline phosphatase and prognostic relevance in adult cardiopulmonary bypass. Interact Cardiovasc Thorac Surg 2020;31:383-90. [Crossref] [PubMed]
- Probstfield JL, Statland BE, Gorman L, et al. Alterations in human serum alkaline phosphatase and its isoenzymes by hypolipidemic agents: colestipol and clofibrate. Metabolism 1983;32:818-21. [Crossref] [PubMed]
- Whitaker KB, Costa D, Moss DW. Selective effects of clofibrate on alkaline phosphatase isoenzymes in serum. Clin Chim Acta 1979;94:191-6. [Crossref] [PubMed]
- Soma K, Izumi M, Yamamoto Y, et al. In Vitro and In Vivo Pharmacological Profiles of DS-1211, a Novel Potent, Selective, and Orally Bioavailable Tissue-Nonspecific Alkaline Phosphatase Inhibitor. J Bone Miner Res 2022;37:2033-43. [Crossref] [PubMed]
- Sajid-Ur-Rehman. Synthesis of sulfadiazinyl acyl/aryl thiourea derivatives as calf intestinal alkaline phosphatase inhibitors, pharmacokinetic properties, lead optimization, Lineweaver-Burk plot evaluation and binding analysis. Bioorg Med Chem 2018;26:3707-15. [Crossref] [PubMed]
- Zizian BV, Gauthier B. Characteristics of the inhibition of serum alkaline phosphatase by theophylline. Clin Biochem 1978;11:57-61. [Crossref] [PubMed]
- Tsiantouli E, Biver E, Chevalley T, et al. Prevalence of Low Serum Alkaline Phosphatase and Hypophosphatasia in Adult Patients with Atypical Femur Fractures. Calcif Tissue Int 2022;110:703-11. [Crossref] [PubMed]
- Rosen CJ, Hochberg MC, Bonnick SL, et al. Treatment with once-weekly alendronate 70 mg compared with once-weekly risedronate 35 mg in women with postmenopausal osteoporosis: a randomized double-blind study. J Bone Miner Res 2005;20:141-51. [PubMed]
- Nakamura Y, Suzuki T, Kato H. Serum bone alkaline phosphatase is a useful marker to evaluate lumbar bone mineral density in Japanese postmenopausal osteoporotic women during denosumab treatment. Ther Clin Risk Manag 2017;13:1343-8. [Crossref] [PubMed]
- Costa-Rodrigues J, Reis S, Teixeira S, et al. Dose-dependent inhibitory effects of proton pump inhibitors on human osteoclastic and osteoblastic cell activity. FEBS J 2013;280:5052-64. [Crossref] [PubMed]
- Metaye T, Mettey Y, Lehuede J, et al. Comparative inhibition of human alkaline phosphatase and diamine oxidase by bromo-levamisole, cimetidine and various derivatives. Biochem Pharmacol 1988;37:4263-8. [Crossref] [PubMed]
- Gill M, Sanyal SN, Sareen ML. Interaction of H2-receptor antagonists, cimetidine and ranitidine with microsomal drug metabolizing and other systems in liver. Indian J Exp Biol 1991;29:852-6. [PubMed]
- Lab Tests Guide. ALP Fractionation Test. [cited 2023 Jul 5]. Available online: https://www.labtestsguide.com/alp-fractionation-test
- Du WX, Duan SF, Chen JJ, et al. Serum bone-specific alkaline phosphatase as a biomarker for osseous metastases in patients with malignant carcinomas: a systematic review and meta-analysis. J Cancer Res Ther 2014;10:C140-3. [Crossref] [PubMed]
- Chinoy MA, Javed MI, Khan A, et al. Alkaline phosphatase as a screening test for osteomalacia. J Ayub Med Coll Abbottabad 2011;23:23-5. [PubMed]
- Shaheen S, Noor SS, Barakzai Q. Serum alkaline phosphatase screening for vitamin D deficiency states. J Coll Physicians Surg Pak 2012;22:424-7. [PubMed]
- Scappaticcio L, Longo M, Maiorino MI, et al. Abnormal Liver Blood Tests in Patients with Hyperthyroidism: Systematic Review and Meta-Analysis. Thyroid 2021;31:884-94. [Crossref] [PubMed]
- Yang M, Zhang L, Huang L, et al. Risk Factors for Elevated Preoperative Alkaline Phosphatase in Patients with Refractory Secondary Hyperparathyroidism. Am Surg 2017;83:1368-72. [Crossref] [PubMed]
- Lieberman D, Phillips D. "Isolated" elevation of alkaline phosphatase: significance in hospitalized patients. J Clin Gastroenterol 1990;12:415-9. [Crossref] [PubMed]
- Han PKJ, Babrow A, Hillen MA, et al. Uncertainty in health care: Towards a more systematic program of research. Patient Educ Couns 2019;102:1756-66. [Crossref] [PubMed]
- Kumar M, Herrera JL. Sarcoidosis and the Liver. Clin Liver Dis 2019;23:331-43. [Crossref] [PubMed]
- De Mulder P, Maertens B, Hoorens A, et al. Extrapulmonary sarcoidosis primarily presenting as cholestatic liver disease. BMJ Case Rep 2019;12:e232618. [Crossref] [PubMed]
- Shipman KE, Holt AD, Gama R. Interpreting an isolated raised serum alkaline phosphatase level in an asymptomatic patient. BMJ 2013;346:f976. [Crossref] [PubMed]
- Lindor KD, Kowdley KV, Harrison ME, et al. ACG Clinical Guideline: Primary Sclerosing Cholangitis. Am J Gastroenterol 2015;110:646-59. [Crossref] [PubMed]
- Kwo PY, Cohen SM, Lim JK. ACG Clinical Guideline: Evaluation of Abnormal Liver Chemistries. Am J Gastroenterol 2017;112:18-35. [Crossref] [PubMed]
- Newsome PN, Cramb R, Davison SM, et al. Guidelines on the management of abnormal liver blood tests. Gut 2018;67:6-19. [Crossref] [PubMed]
- van Ooteghem NA, Klomp LW, van Berge-Henegouwen GP, et al. Benign recurrent intrahepatic cholestasis progressing to progressive familial intrahepatic cholestasis: low GGT cholestasis is a clinical continuum. J Hepatol 2002;36:439-43. [Crossref] [PubMed]
- Chapman MH, Thorburn D, Hirschfield GM, et al. British Society of Gastroenterology and UK-PSC guidelines for the diagnosis and management of primary sclerosing cholangitis. Gut 2019;68:1356-78. [Crossref] [PubMed]
- EASL Clinical Practice Guidelines. management of cholestatic liver diseases. J Hepatol 2009;51:237-67. [Crossref] [PubMed]
- Hirschfield GM, Dyson JK, Alexander GJM, et al. The British Society of Gastroenterology/UK-PBC primary biliary cholangitis treatment and management guidelines. Gut 2018;67:1568-94. [Crossref] [PubMed]
- Myszor M, James OF. The epidemiology of primary biliary cirrhosis in north-east England: an increasingly common disease? Q J Med 1990;75:377-85. [PubMed]
- Lindor KD, Bowlus CL, Boyer J, et al. Primary Biliary Cholangitis: 2018 Practice Guidance from the American Association for the Study of Liver Diseases. Hepatology 2019;69:394-419. [Crossref] [PubMed]
- Kaplan MM, Gershwin ME. Primary biliary cirrhosis. N Engl J Med 2005;353:1261-73. [Crossref] [PubMed]
- Nakamura M, Kondo H, Mori T, et al. Anti-gp210 and anti-centromere antibodies are different risk factors for the progression of primary biliary cirrhosis. Hepatology 2007;45:118-27. [Crossref] [PubMed]
- Maggs JR, Chapman RW. An update on primary sclerosing cholangitis. Curr Opin Gastroenterol 2008;24:377-83. [Crossref] [PubMed]
- Hirschfield GM, Gershwin ME. The immunobiology and pathophysiology of primary biliary cirrhosis. Annu Rev Pathol 2013;8:303-30. [Crossref] [PubMed]
- Chapman RW, Arborgh BA, Rhodes JM, et al. Primary sclerosing cholangitis: a review of its clinical features, cholangiography, and hepatic histology. Gut 1980;21:870-7. [Crossref] [PubMed]
- Boberg KM, Fausa O, Haaland T, et al. Features of autoimmune hepatitis in primary sclerosing cholangitis: an evaluation of 114 primary sclerosing cholangitis patients according to a scoring system for the diagnosis of autoimmune hepatitis. Hepatology 1996;23:1369-76. [Crossref] [PubMed]
- Mendes FD, Jorgensen R, Keach J, et al. Elevated serum IgG4 concentration in patients with primary sclerosing cholangitis. Am J Gastroenterol 2006;101:2070-5. [Crossref] [PubMed]
- Björnsson E, Chari ST, Smyrk TC, et al. Immunoglobulin G4 associated cholangitis: description of an emerging clinical entity based on review of the literature. Hepatology 2007;45:1547-54. [Crossref] [PubMed]
- Björnsson E, Chari S, Silveira M, et al. Primary sclerosing cholangitis associated with elevated immunoglobulin G4: clinical characteristics and response to therapy. Am J Ther 2011;18:198-205. [Crossref] [PubMed]
- Hov JR, Boberg KM, Karlsen TH. Autoantibodies in primary sclerosing cholangitis. World J Gastroenterol 2008;14:3781-91. [Crossref] [PubMed]
- Corpechot C, El Naggar A, Poujol-Robert A, et al. Assessment of biliary fibrosis by transient elastography in patients with PBC and PSC. Hepatology 2006;43:1118-24. [Crossref] [PubMed]
- Exeter Laboratory. Alkaline Phosphatase (ALP). 2019. [cited 2023 Jul 5]. Available online: https://www.exeterlaboratory.com/test/alkaline-phosphatase/
- McPherson GS, Jani BR. Isolated increase in serum alkaline phosphatase with sodium valproate therapy. Ann Pharmacother 1995;29:539. [Crossref] [PubMed]
- Cepelak I, Rekić B, Juretić D, et al. Effect of sodium valproate on renal cell brush-border enzymes in rats. Eur J Clin Chem Clin Biochem 1995;33:673-7. [Crossref] [PubMed]
- Burgunder JM, Abernethy DR, Lauterburg BH. Liver injury due to verapamil. Hepatogastroenterology 1988;35:169-70. [PubMed]
- Iqbal U, Siddiqui HU, Anwar H, et al. Allopurinol-Induced Granulomatous Hepatitis: A Case Report and Review of Literature. J Investig Med High Impact Case Rep 2017;5:2324709617728302. [Crossref] [PubMed]
- Math MV. Furosemide and increased serum alkaline phosphatase (hepatic isoenzyme). Clin Chem 1982;28:1812-3. [Crossref] [PubMed]
- Kwda A, Gldc P, Baui B, et al. Effect of long term inhaled corticosteroid therapy on adrenal suppression, growth and bone health in children with asthma. BMC Pediatr 2019;19:411. [Crossref] [PubMed]
- Chamani S, Liberale L, Mobasheri L, et al. The role of statins in the differentiation and function of bone cells. Eur J Clin Invest 2021;51:e13534. [Crossref] [PubMed]
- Boks MN, Tiebosch AT, van der Waaij LA. A jaundiced bodybuilder Cholestatic hepatitis as side effect of injectable anabolic-androgenic steroids. J Sports Sci 2017;35:2262-4. [Crossref] [PubMed]
- Magnusson P, Häger A, Larsson L. Serum osteocalcin and bone and liver alkaline phosphatase isoforms in healthy children and adolescents. Pediatr Res 1995;38:955-61. [Crossref] [PubMed]
- Nizet A, Cavalier E, Stenvinkel P, et al. Bone alkaline phosphatase: An important biomarker in chronic kidney disease - mineral and bone disorder. Clin Chim Acta 2020;501:198-206. [Crossref] [PubMed]
- Uday S, Högler W. Rickets and Osteomalacia. Encyclopedia of Endocrine Diseases 2019;5:339-54.
- Whyte MP, Thakker RV. Rickets and osteomalacia. Medicine 2013;41:594-9. [Crossref] [PubMed]
- Magne D, Bluteau G, Faucheux C, et al. Phosphate is a specific signal for ATDC5 chondrocyte maturation and apoptosis-associated mineralization: possible implication of apoptosis in the regulation of endochondral ossification. J Bone Miner Res 2003;18:1430-42. [Crossref] [PubMed]
- Sabbagh Y, Carpenter TO, Demay MB. Hypophosphatemia leads to rickets by impairing caspase-mediated apoptosis of hypertrophic chondrocytes. Proc Natl Acad Sci U S A 2005;102:9637-42. [Crossref] [PubMed]
- Ralston SH, Langston AL, Reid IR. Pathogenesis and management of Paget's disease of bone. Lancet 2008;372:155-63. [Crossref] [PubMed]
- Reid IR. Recent advances in understanding and managing Paget's disease. F1000Res 2019;8:F1000 Faculty Rev-1485.
- Alvarez L, Peris P, Pons F, et al. Relationship between biochemical markers of bone turnover and bone scintigraphic indices in assessment of Paget's disease activity. Arthritis Rheum 1997;40:461-8. [Crossref] [PubMed]
- Delmas PD, Meunier PJ. The management of Paget's disease of bone. N Engl J Med 1997;336:558-66. [Crossref] [PubMed]
- Corral-Gudino L, Tan AJ, Del Pino-Montes J, et al. Bisphosphonates for Paget's disease of bone in adults. Cochrane Database Syst Rev 2017;12:CD004956. [Crossref] [PubMed]
- Langston AL, Campbell MK, Fraser WD, et al. Randomized trial of intensive bisphosphonate treatment versus symptomatic management in Paget's disease of bone. J Bone Miner Res 2010;25:20-31. [Crossref] [PubMed]
- Tan A, Goodman K, Walker A, et al. Long-Term Randomized Trial of Intensive Versus Symptomatic Management in Paget's Disease of Bone: The PRISM-EZ Study. J Bone Miner Res 2017;32:1165-73. [Crossref] [PubMed]
- Singer FR. The evaluation and treatment of Paget's disease of bone. Best Pract Res Clin Rheumatol 2020;34:101506. [Crossref] [PubMed]
- Kling JM, Clarke BL, Sandhu NP. Osteoporosis prevention, screening, and treatment: a review. J Womens Health (Larchmt) 2014;23:563-72. [Crossref] [PubMed]
- Nakagawa H, Kamimura M, Takahara K, et al. Changes in total alkaline phosphatase level after hip fracture: comparison between femoral neck and trochanter fractures. J Orthop Sci 2006;11:135-9. [Crossref] [PubMed]
- Dresner-Pollak R, Mayer M, Hochner-Celiniker D. The decrease in serum bone-specific alkaline phosphatase predicts bone mineral density response to hormone replacement therapy in early postmenopausal women. Calcif Tissue Int 2000;66:104-7. [Crossref] [PubMed]
- Cheng SL, Yang JW, Rifas L, et al. Differentiation of human bone marrow osteogenic stromal cells in vitro: induction of the osteoblast phenotype by dexamethasone. Endocrinology 1994;134:277-86. [Crossref] [PubMed]
- Hardy RS, Zhou H, Seibel MJ, et al. Glucocorticoids and Bone: Consequences of Endogenous and Exogenous Excess and Replacement Therapy. Endocr Rev 2018;39:519-48. [Crossref] [PubMed]
- Hyldstrup L, Clemmensen I, Jensen BA, et al. Non-invasive evaluation of bone formation: measurements of serum alkaline phosphatase, whole body retention of diphosphonate and serum osteocalcin in metabolic bone disorders and thyroid disease. Scand J Clin Lab Invest 1988;48:611-9. [Crossref] [PubMed]
- Mikosch P. Effects of thyroid disorders on the bone. Wien Med Wochenschr 2005;155:444-53. [Crossref] [PubMed]
- Lehmann FG. Immunological methods for human placental alkaline phosphatase (Regan isoenzyme). Clin Chim Acta 1975;65:271-82. [Crossref] [PubMed]
- Moss DW. Diagnostic aspects of alkaline phosphatase and its isoenzymes. Clin Biochem 1987;20:225-30. [Crossref] [PubMed]
- Hada T, Higashino K, Okochi T, et al. Kasahara-variant alkaline phosphatase in a renal cell carcinoma. Clin Chim Acta 1978;89:311-6. [Crossref] [PubMed]
- Wei SC, Doellgast GJ. Immunochemical studies of human placental-type variants of alkaline phosphatase. Structural differences between the "Nagao isoenzyme" and the placental "D-variant". Eur J Biochem 1981;118:39-45. [Crossref] [PubMed]
- Behúlová D, Bzdúch V, Holesová D, et al. Transient hyperphosphatasemia of infancy and childhood: study of 194 cases. Clin Chem 2000;46:1868-9. [Crossref] [PubMed]
- Gualco G, Lava SA, Garzoni L, et al. Transient benign hyperphophatasemia. J Pediatr Gastroenterol Nutr 2013;57:167-71. [Crossref] [PubMed]
- Stein P, Rosalki SB, Foo AY, et al. Transient hyperphosphatasemia of infancy and early childhood: clinical and biochemical features of 21 cases and literature review. Clin Chem 1987;33:313-8. [Crossref] [PubMed]
- Huh SY, Feldman HA, Cox JE, et al. Prevalence of transient hyperphosphatasemia among healthy infants and toddlers. Pediatrics 2009;124:703-9. [Crossref] [PubMed]
- O'Riordan S, Baker AJ, Sherwood RA. Isoenzyme characterization in isolated elevation of alkaline phosphatase after liver transplantation in children. Transplantation 2002;74:1030-4. [Crossref] [PubMed]
- Sampani E, Kasimatis E, Memmos E, et al. Transient Hyperphosphatasemia in an Adolescent and an Adult Renal Transplant Patient. Transplant Proc 2021;53:2769-70. [Crossref] [PubMed]
- Maldonado O, Demasi R, Maldonado Y, et al. Extremely high levels of alkaline phosphatase in hospitalized patients. J Clin Gastroenterol 1998;27:342-5. [Crossref] [PubMed]
- Aida S. Alkaline phosphatase isoenzyme activities in rheumatoid arthritis: hepatobiliary enzyme dissociation and relation to disease activity. Ann Rheum Dis 1993;52:511-6. [Crossref] [PubMed]
- Siede WH, Seiffert UB, Merle S, et al. Alkaline phosphatase isoenzymes in rheumatic diseases. Clin Biochem 1989;22:121-4. [Crossref] [PubMed]
- Ryu WS, Lee SH, Kim CK, et al. High serum alkaline phosphatase in relation to cerebral small vessel disease. Atherosclerosis 2014;232:313-8. [Crossref] [PubMed]
- Kang KY, Hong YS, Park SH, et al. Increased serum alkaline phosphatase levels correlate with high disease activity and low bone mineral density in patients with axial spondyloarthritis. Semin Arthritis Rheum 2015;45:202-7. [Crossref] [PubMed]
- Cho SR, Lim YA, Lee WG. Unusually high alkaline phosphatase due to intestinal isoenzyme in a healthy adult. Clin Chem Lab Med 2005;43:1274-5. [Crossref] [PubMed]
- Bayer PM, Hotschek H, Knoth E. Intestinal alkaline phosphatase and the ABO blood group system--a new aspect. Clin Chim Acta 1980;108:81-7. [Crossref] [PubMed]
- Matsushita M, Harajiri S, Tabata S, et al. Alkaline phosphatase activity in blood group B or O secretors is fluctuated by the dinner intake of previous night. Rinsho Byori 2013;61:307-12. [PubMed]
- Verma J, Gorard DA. Persistently elevated alkaline phosphatase. BMJ Case Rep 2012;2012:bcr2012006768. [Crossref] [PubMed]
- Rosalki SB, Foo AY, Dooley JS. Benign familial hyperphosphatasaemia as a cause of unexplained increase in plasma alkaline phosphatase activity. J Clin Pathol 1993;46:738-41. [Crossref] [PubMed]
- Anh DJ, Eden A, Farley JR. Quantitation of soluble and skeletal alkaline phosphatase, and insoluble alkaline phosphatase anchor-hydrolase activities in human serum. Clin Chim Acta 2001;311:137-48. [Crossref] [PubMed]
- Magnusson P, Sharp CA, Farley JR. Different distributions of human bone alkaline phosphatase isoforms in serum and bone tissue extracts. Clin Chim Acta 2002;325:59-70. [Crossref] [PubMed]
- Zierk J, Arzideh F, Haeckel R, et al. Pediatric reference intervals for alkaline phosphatase. Clin Chem Lab Med 2017;55:102-10. [Crossref] [PubMed]
- Colantonio DA, Kyriakopoulou L, Chan MK, et al. Closing the gaps in pediatric laboratory reference intervals: a CALIPER database of 40 biochemical markers in a healthy and multiethnic population of children. Clin Chem 2012;58:854-68. [Crossref] [PubMed]
- Zhan F, Watanabe Y, Shimoda A, et al. Evaluation of serum bone alkaline phosphatase activity in patients with liver disease: Comparison between electrophoresis and chemiluminescent enzyme immunoassay. Clin Chim Acta 2016;460:40-5. [Crossref] [PubMed]
- Curtis EM, Parsons C, Maslin K, et al. Bone turnover in pregnancy, measured by urinary CTX, is influenced by vitamin D supplementation and is associated with maternal bone health: findings from the Maternal Vitamin D Osteoporosis Study (MAVIDOS) trial. Am J Clin Nutr 2021;114:1600-11. [Crossref] [PubMed]
- Walker I, Chappell LC, Williamson C. Abnormal liver function tests in pregnancy. BMJ 2013;347:f6055. [Crossref] [PubMed]
Cite this article as: Irving M, Shipman AR, Shipman KE. Investigative algorithms for disorders affecting human plasma alkaline phosphatase: a narrative review. J Lab Precis Med 2024;9:7.