Clinical evaluation of hyperferritinemia with or without iron overload
Introduction
In clinical practice, physicians order serum ferritin assay as a useful biomarker in cases of iron deficiency anemia (low serum ferritin) or iron overload (high serum ferritin). Besides iron overload, high serum ferritin is caused by a non-iron overload condition, such as viral [Epstein Barr virus, severe acute respiratory syndrome coronavirus 2 (SARS-CoV-2) infections, etc.] or bacterial infectious diseases (sepsis) or inflammatory diseases (Still’s disease, juvenile acute arthritis, etc.), in which serum ferritin levels are employed to evaluate disease activity/severity. Most strikingly, when such conditions progress to hemophagocytic lymphohistiocytosis (HLH) or macrophage activation syndrome (MAS), a marked increase in serum ferritin develops. In addition, it is not infrequently that metabolic patients with hyperferritinemia are referred to hematologists who are not familiar with metabolic cases. Although the incidence is very low, physicians need to be aware of Hereditary Hyperferritinemia-Cataract Syndrome (HHCS) and other hereditary hyperferritinemia. In this review, differential diagnosis of various causes of hyperferritinemia with or without iron overload, characteristics of serum ferritin, and management have been overviewed.
Characteristics of serum ferritin
Iron homeostasis and ferritin
Hyperferritinemia is largely related to disturbed iron homeostasis. Iron homeostasis relies on the amount of iron absorbed by the intestine and its release from storage sites and is also dependent on the amount of iron used for erythropoiesis. In iron metabolism, many molecules are involved such as divalent metal-ion transporter 1 (DMT1), HFE, hepcidin, ferroportin 1, transferrin, ceruloplasmin, transferrin receptor, hemojuvelin, etc. of which hepcidin plays the main regulator (1). Decreased hepcidin synthesis or disrupted hepcidin binding to ferroportin causes iron overload and hyperferritinemia. Within the cells where iron is transferred, iron regulatory proteins (IRPs) bind to the iron-responsive element (IRE). The IRE/IRP interaction and hepcidin are coordinated with the fluctuation of the cellular iron level (2). There is also a link between iron and copper metabolism. Ceruloplasmin, which contains greater than 95% of the copper found in plasma, is also a player in iron metabolism as ferroxidase that oxidizes toxic ferrous iron (Fe2+) to its nontoxic ferric form (Fe3+), which is then taken up by transferrin. Thus, in daily practice, abnormal serum values of ferritin, hepcidin, transferrin, and ceruloplasmin are noted in diseases of disturbed iron homeostasis. Hyperferritinemia occurs in various pathological conditions with or without iron overload (3,4). Ferritin, with a molecular weight of approximately 500 kDa, is the major iron-storage glycoprotein found in all tissues. It is comprised of 24 subunits containing H-chain (heavy or heart, 21 kDa), and L-chain (light or liver, 19 kDa) polypeptide chains encoded by two different genes; H- and L-ferritin (FTH and FTL) genes located on chromosomes 11q and 19q (5,6). Thus, ferritin molecules are heteropolymers of H- and L-ferritin and do not function as homopolymers. In terms of intracellular localization of ferritin subunits, L-ferritin is predominantly found in the cytosol, while H-ferritin is mainly found in the nucleus. Ferritin polymers rich in L-ferritin play more of a storage function, whereas those rich in H-ferritin may have functions beyond iron storage (6). Ferritin synthesis is regulated by cytokines [tumor necrosis factor (TNF)-alpha and interleukin-1 alpha] at various levels (transcriptional, post-transcriptional, translational) during development, cellular differentiation, proliferation, and inflammation (7-9). Serum ferritin comprises mostly L-ferritin subunits (5,6). Also, ferritin resides in glycosylated and non-glycosylated forms (6,10,11). From the clinical point of view, an assay of serum ferritin is essential, and the glycosylation status of ferritin could be useful for the differential diagnosis of hyperferritinemia, though the glycosylation status remains a research test and is not routinely utilized in clinical practice.
Source and characteristics of serum ferritin
There are two probable mechanisms as the source of increased serum ferritin; one is that it arises from damaged cells, representing a marker of cellular damage (12,13). Increased ferritin release from the injured cells may occur due to chronic viral hepatitis, metabolic hepatic steatosis (see metabolic hyperferritinemia), etc. (13). On the other hand, increased ferritin synthesis can be noted most strikingly in cases of infectious/inflammatory hyperferritinemia, which is caused by a rapid release along the augmented synthesis, not by leakage from damaged cells (14). In a mouse model, ferritin is secreted as an acute reactant from cells such as splenic macrophages, hepatocytes, Kupffer cells, and proximal tubular renal cells where ferritin is rapidly synthesized under the effect of cytokines (15). Thus, serum ferritin as a well-known acute-phase reactant reflects the degree of acute and chronic infectious/inflammatory diseases (16). In hemochromatosis and inherited iron overloading anemias, increased ferritin synthesis was also suggested (13). Among the cases of hereditary hyperferritinemia, in HHCS cases, it is due to constitutive L-ferritin production (17). In FTL gene mutation cases, an abnormal ferritin secretion is hypothesized but not confirmed (18). In cases of STAB1 gene mutation cases, the mechanism remains to be clarified (19). Serum ferritin is glycosylated (normally ~50%), but there are two pathological conditions, with low glycosylated (20–42%), or hyperglycosylated (>90%) as discussed later.
Definition of hyperferritinemia
Normal values of serum ferritin differ from laboratory to laboratory. We use 5–157 ng/mL as normal ranges in our laboratory. Kowdley et al. employed >1.5 × upper limit of normal (ULN; >300 ng/mL in women and >450 ng/mL in men) as a definition of hyperferritinemia in metabolic diseases (20). If this definition is adapted for our laboratory >236 ng/mL could be hyperferritinemia regardless of males or females. On the other hand, diagnostic criteria for HLH and MAS employed ≥500 and >684 ng/mL, respectively, as hyperferritinemia (21,22). Hyperferritinemia could be classified from mild (>1.5 × ULN–500 ng/mL), moderate (500–3,000 ng/mL), and markedly high (3,000–>100,000 ng/mL). Thus, as defining hyperferritinemia, strict cut-off values such as >1.5 × UNL are employed for mild~moderate increase while a cut-off of ≥500–684 ng/mL could be employed for a disease showing markedly high serum ferritin.
Differentiation of hyperferritinemia with or without iron overload
As mentioned above, hyperferritinemia does not necessarily mean iron overload. To differentiate if hyperferritinemia is associated with or without iron overload, serum iron concentration as well as transferrin saturation (TSAT; calculated as serum iron/total iron binding capacity × 100), are good indicators for this purpose. In addition, magnetic resonance imaging (MRI) of the liver is useful, because whenever iron overload is present, the liver is the main organ involved. For directly assessing the liver iron content (LIC), liver biopsy was previously employed as the standard procedure but is less used than before because the high level of precision of T2* measurement by dedicated MRI now allows a non-invasive and efficient procedure for measurement of iron concentration in the liver and other organs (23,24).
Major causes of hyperferritinemia
Major causes of hyperferritinemia and their characteristics are shown as an algorithm in Table 1 and Figure 1, and the degree of serum ferritin levels are comparatively illustrated in Figure 2. Hyperferritinemia-related gene panels due to inherited disorders (25,26) are listed in Table 2. Below, each hyperferritinemic disease is overviewed in the order of non-iron overload, mixed type (with or without iron overload), and iron overload.
Table 1
Diseases | Causes | Hyperferritinemia** | Iron overload |
---|---|---|---|
Infectious disease/autoimmune disease-triggered | No HLH/MAS | Mild to moderate | No |
HLH*/MAS | Marked | ||
Hereditary hyperferritinemia | HHCS | Mild to moderate | No |
FTL gene mutation | Mild to marked | ||
STAB1 gene mutation | Mild to moderate | ||
Metabolic syndrome | NAFLD | Mild | Mixed |
DIOS | Mild to moderate | ||
Hemochromatosis | HFE/non-HFE | Mild to moderate | Yes |
Inherited iron overloading anemia | DHS1 | Mild to marked | Yes |
PKD | |||
XLAS | |||
CDA | |||
SCD | |||
Thalassemia | |||
Other rare disorders causing iron overload | Aceruloplasminemia | Mild to moderate | Yes |
Atransferrinemia | |||
DMT1 deficiency |
Inherited iron overloading anemia is caused by hemolysis, ineffective erythropoiesis inhibiting hepcidin synthesis, and increasing iron absorption and release in the blood. In severe anemic cases, transfusion-related iron overload is also responsible. *, there are two types of HLH; primary (inherited; see Table 2) and secondary; **, increase of ferritin: mild increase (>1.5 × ULN–500 ng/mL), moderate increase (>500–3,000 ng/mL) and marked increase (3,000−>100,000 ng/mL). HLH, hemophagocytic lymphohistiocytosis; MAS, macrophage activation syndrome; HHCS, Hereditary hyperferritinemia due to cataract syndrome; FTL gene, L-ferritin gene; STAB1, encoding stabilin 1 protein; NAFLD, non-alcoholic fatty liver disease; DIOS, dysmetabolic iron overload syndrome; DHS1, dehydrated hereditary stomatocytosis 1; PKD, pyruvate kinase deficiency; XLAS, X-linked sideroblastic anemia; CDA, congenital dyserythropoietic anemia; SCD, sickle cell disease; DMT1, divalent metal-ion transporter 1.
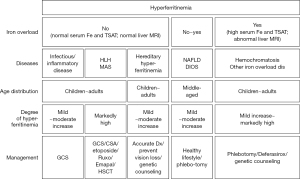
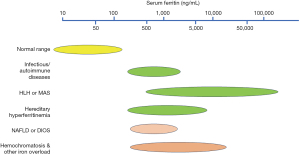
Table 2
Primary HLH and related | Hemochromatosis | Hereditary hyperferritinemia | Inherited iron-overloading anemias | |
---|---|---|---|---|
FRF1 | HFE | IRE region of FLT | PIEZO1 | CP |
UNC13D | HAMP | FLT | PKLR | TF |
STX11 | TFR2 | STAB1 | ALAS2 | DMT1 (SCL11A2) |
STXB2 | SLC40A1 | CDAN1 | *TMPRSS6 | |
LYST | KLF1 | |||
RAB27A | SEC23B | |||
ADTB3A | Thalassemia gene | |||
SH2D1A | SCA beta-globin gene | |||
XIAP |
Hyperferritinemia in infectious/ inflammatory diseases
This condition is noted from young children to the elderly. Causes of hyperferritinemia are due to acute ferritin synthesis under the cytokine effect. The cellular response by cytokines to viral and bacterial infections, or inflammatory diseases like pediatric systemic lupus erythematosus (SLE), systemic juvenile idiopathic arthritis (sJIA), or adult-onset Still’s disease (AOSD) stimulates the expression of ferritin genes (16,27-30). High serum ferritin levels have been described in viral infectious conditions, such as Epstein-Barr virus (EBV) (31-33) and SARS-CoV-2 infections, etc. (34-36). Particularly, during the recent pandemic of SARS-CoV-2 infection, data on coronavirus disease 2019 (COVID-19)-triggered hyperferritinemia has been accumulated (34-36), in which the serum ferritin levels in severe COVID-19 patients were significantly increased compared with the levels in non-severe patients (34). Even in sepsis, a high level of serum ferritin was an independent prognostic marker for the prediction of mortality (37,38). In infectious/inflammatory diseases, serum ferritin, as one of the key acute-phase reactants, is employed to evaluate disease activity/severity if the disease develops into HLH or MAS. For the diagnosis of HLH/MAS, physicians carefully must refer to the diagnostic criteria of HLH (21) or MAS (22,39). It is well-recognized that the laboratory abnormalities in HLH and MAS overlap. Actual serum levels of ferritin were; in EBV-infectious mononucleosis >1,650 ng/mL (33), 2,430 ng/mL (31), and 1,901 ng/mL (32). In patients with COVID-19, serum ferritin values were median (ranges) of 1,024 (434–1,821) ng/mL, and a median of 501.9 ng/mL in the survivor group vs. 1,722 ng/mL in the non-survivor group (35). In patients with sepsis, serum ferritin values were median (ranges) of 542 (244–1,125) ng/mL (38), and a median of 430 ng/mL in the survivor group vs. 892 ng/mL in the non-survivor group (37). In the management of these cases, glucocorticoids (GCS) or cyclosporine A (CSA) are mostly effective (27). For cases of COVID-19 with severe systemic hyperinflammation, the Janus kinase 1/2 inhibitor ruxolitinib has been employed (40).
Hyperferritinemia in HLH, or MAS
HLH or MAS develops from the above infectious/ inflammatory diseases, which fulfill the diagnostic criteria of either HLH or MAS and occur in young children to the elderly. HLH is classified as primary (hereditary) and secondary (21,25), while MAS is secondary under inflammatory diseases, as mentioned above. Historically, when we first noted significantly higher levels of serum ferritin in cases of hemophagocytic syndrome in the mid-1980s (41), on a literature survey such high values were only known in cases of Lysinuric Protein Intolerance (LPI), a metabolic disease (42), which was later clarified caused by HLH. To date, significant amounts of data on this type of hyperferritinemia have been accumulated and a marked increase of serum ferritin is well recognized in HLH (43-45) and MAS associated with pediatric sJIA or with SLE (22, 29,39). Hyperferritinemia in this category indicates the activation of the monocyte-macrophage system, which is a crucial part of the inflammatory cytokine storm. In the differentiation of hyperferritinemia between HLH or MAS and hemochromatosis, it was once proposed that evaluating clinical characteristics, serum triglycerides, and blood counts was the only reliable and rapid way to distinguish the two conditions (46). However, more recently, Ishihara et al. proposed that glycosylated ferritin could be an improved differential marker between these two hyperferritinemia (47). A low percentage (<20%) of glycosylated ferritin has been reported as a marker of HLH or MAS (48-50). As above mentioned, the highly elevated serum ferritin in HLH or MAS cases is due to a rapidly increased synthesis under the effect of cytokines such as TNF-alpha (8). Thus, markedly high serum ferritin in HLH or MAS is not normally (~50%) glycosylated. Actual serum ferritin levels of HLH reported were; a maximum value ranging from 1,140 to 68,600 ng/mL (43), and in hospitalized cases, on admission from 757 to 63,919 ng/mL, and a maximum from 994 to 189,721 ng/mL (44). In MAS cases, serum ferritin values were; median (ranges) of 7,838 (360–150,099) ng/mL in sJIA-MAS, and 4,158 (1,300–15,456) ng/mL in SLE-MAS (29). These data indicate that serum ferritin values in HLH or MAS are much higher, compared to mild to moderate increases in other diseases showing hyperferritinemia (Figure 2). As management, most HLH and MAS cases could be controlled with GCS and CSA with/without etoposide (27). HLH-94 or HLH-2004 regimen (dexamethasone/CSA/etoposide) has most commonly and globally been employed to date (21,51). More recently, ruxolitinib (52-54) and emapalumab (a fully human anti-IFNγ monoclonal antibody) (55-58) have proven to be effective in the control of disease activity. However, hereditary HLH requires hematopoietic stem cell transplantation (59).
Hereditary hyperferritinemia: HHCS and others
Hereditary hyperferritinemia is extremely rare and has been reported without iron overload in otherwise healthy individuals. There are three types of hereditary hyperferritinemia reported. One type is HHCS which is an autosomal dominant inheritance disease characterized by congenital bilateral cataracts associated with high serum ferritin levels. In HHCS, hyperferritinemic children aged 7–9 years can be diagnosed with a positive family history of early onset cataracts, who have no tissue iron overload or inflammation (17,60,61). The cause of hyperferritinemia in HHCS is the dysregulation of the IRE/IRP system due to mutations in the IRE stem-loop, i.e., the gene mutations are detected in IRE in the 5' untranslated region of the L ferritin mRNA, and L-ferritin synthesis is constitutively upregulated (62) which is responsible for the increase of serum low glycosylated (20–40%) serum ferritin (17,62). L-ferritin aggregates accumulate preferentially in the lens, provoking bilateral cataracts since childhood (63). The second type of hereditary hyperferritinemia is caused by a novel heterozygous p.Thr30Ile mutation in the NH2 terminus of L-ferritin subunit (“A” alpha helix) identified by sequencing analysis of the ferritin light chain (FTL) gene. This mutation is hypothesized to increase the efficacy of L-ferritin secretion into sera due to the increased hydrophobicity of the N terminal (18). Kannengiesser et al. identified this mutation in 25 family cases and 66 isolated cases, aged from 8 to 83 years (18). Similarly, two novel missense L-ferritin variants; p.Gln26Ile and p.Ala27Val. were also reported (64). These cases were 45- and 75-year-old. In this FTL gene mutated cases, hyperglycosylated (>90%) ferritin is characteristic (18,64). As another genetic cause of hereditary hyperferritinemia, ten subjects from seven families due to bi-allelic STAB1 (encoding stabilin-1) mutations were described, suggesting an important role of stabilin-1 in the regulation of serum ferritin levels (19). Actual serum ferritin values reported in HHCS were; from 366 to 1,890 ng/mL (60), from 239 to 1,290 ng/mL (61), from 919 to 1,143 ng/mL (17), and from 653 to 1,796 ng/mL (63). Serum ferritin values in the disease of FTL gene mutations were; from 400 to 6,000 ng/mL (18) and from 618 to 1,200 ng/mL (64), and in the STAB1 mutation disease were; median (ranges) 1,970 ng/mL (365–4,654) ng/mL (19). In the management of hereditary hyperferritinemia, accurate diagnosis is essential to avoid unnecessary treatment and to prevent early vision loss in HHCS. In addition, genetic counseling is required for other family members who are at risk (65).
Metabolic hyperferritinemia with or without iron overload:
It is well known that mild hyperferritinemia is observed in one-third of patients with non-alcoholic fatty liver disease (NAFLD) (66-69) or patients of dysmetabolic iron overload syndrome (DIOS), which is also termed Dysmetabolic Hepatic Iron Overload (DHIO) (70-73). These patients exhibit mildly elevated serum ferritin associated with high serum alanine aminotransferase due to liver steatosis and high fasting insulin C-peptide due to insulin resistance. The diagnosis of metabolic hyperferritinemia is mainly made in middle-aged males, associated with mostly low to normal TSAT (22–45%) and with various metabolic abnormalities, such as increased body mass index with android fat distribution, elevated blood pressure, dyslipidemia, abnormal glucose metabolism, steatohepatitis (66). Though iron overload is not noted in all cases, DIOS cases mostly show mild hepatic iron excess in MRI studies which needs to be differentiated from ferroportin disease (74). In cases where phlebotomy was employed, normalized liver dysfunction and insulin resistance associated with decreased ferritin levels could be obtained (67,68). However, its pathophysiology and the degree to which it reflects tissue iron overload remain unclear. In NAFLD or DIOS, serum or urinary hepcidin levels are higher, indicating that synthesis of this hormone is not impaired, which thus is not responsive to iron storage (75,76). One hypothesis is that iron accumulation in metabolic diseases is due to the inhibition of iron mobilization from hepatocytes and Kupffer cells (66,67,69). Accordingly, the pathogenesis of hyperferritinemia in these cases seems to differ from that in hemochromatosis, in which serum or urinary hepcidin is impaired. Nevertheless, the increased production of hepcidin in patients with NAFLD or with DIOS is not yet fully understood (70). More recently, metabolic hyperferritinemia is classified as Stage 1 (normal iron stores, serum ferritin <550 ng/mL), Stage 2 (increased iron stores, serum ferritin 550–1,000 ng/mL), and Stage 3 (DIOS; very increased iron stores, serum ferritin >1,000 ng/mL) (77). Actual serum ferritin values reported in the NAFLD were; from 366 to 1,080 ng/mL (66), from 283 to 2,190 ng/mL (68), and a case of DIOS showed 2,210 ng/mL (74). As management, because high serum ferritin and hepatic iron stores are associated with the risk of several liver diseases, type 2 diabetes mellitus, and cardiovascular damage, a healthy lifestyle, such as a balanced diet low in processed foods, regular exercise, and limited alcohol consumption, and the pharmacological control of cardiovascular risk factors are recommended (77). In addition, phlebotomy can be employed for cases with iron overload (67,68).
Hyperferritinemia in hemochromatosis
Hemochromatosis was previously classified as primary (hereditary) and secondary (78,79). However, more recently, hemochromatosis has been defined as a non-anemic group of inherited disorders that cause iron overload due to failed regulation of hepcidin (26,80,81). Patients with iron overload including hemochromatosis show high serum Fe levels, TSAT, and abnormal images [computed tomography (CT), MRI] of the liver (23,24,26,80-82). Clinically, patients with iron overload initially may be asymptomatic, but if progressed it presents with hepatic dysfunction, endocrine abnormalities like diabetes mellitus or hypogonadism, heart failure, CNS diseases, and other organ problems. Precise diagnosis of hemochromatosis, and other iron-overloading anemias may require genetic studies (26,82) (Table 2, Figure 3). In Japan, of 1,109 iron overload cases analyzed, the number of hemochromatosis was very limited, and the remaining 1,033 cases (93.1%) were transfusion-related (82). Hemochromatosis could have an autosomal recessive inheritance (HFE type 1 as well as non-HFE types 2 to 4) (80). In Caucasians, type 1 could be the major type, where HFE homozygous (C282Y) gene mutation is the most common in whites (83,84). On the other hand, in other ethnic groups, non-HFE types may be prevalent (85). Non-HFE-types consist of mutations in the four main genes: hemojuvelin (HJV, type 2A juvenile hemochromatosis), hepcidin (HAMP, type 2B juvenile hemochromatosis), transferrin receptor 2 (TFR2, type 3 hemochromatosis), and type 4 hemochromatosis (SLC40A1, previous type 4B) (85-89). Gene mutations of SLC40A1 encoding ferroportin-the unique cellular iron exporter, whose function is controlled by hepcidin, cause two types of iron overload disease. One is ferroportin disease associated with mild iron overload, which is due to "loss of function" mutations causing iron retention in reticuloendothelial cells and hyperferritinemia with normal TSAT (previous type 4A). The other is type 4 hemochromatosis, caused by "gain of function" mutations associated with severe iron overload, showing resistance to hepcidin-mediated ferroportin degradation (26). Currently, ferroportin disease is no more included in hemochromatosis. Actual serum ferritin values in hemochromatosis were; from 200 to 1,000 ng/mL in type 1 (84), 2,222–16,000 ng/mL in 4 cases of type 2A (85,88), 3,000 and 5,696 ng/mL in two cases of type 2B (85,89), from 1,057 to 10,191 ng/mL in 5 cases of type 3 (85), and 7,980 ng/mL (85), >1,650 and 10,175 ng/mL (87) in 3 cases of type 4 hemochromatosis. As management, periodic phlebotomy, and oral chelating agent deferasirox are optionally employed. It is recommended that serum ferritin needs to be reduced to 50–100 ng/mL (90). In addition, genetic counseling is required for any patients with hemochromatosis.
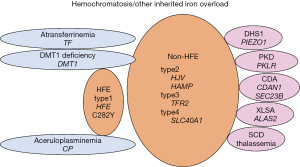
Other iron overloading anemias
Other inherited iron-loading anemias are characterized by ineffective erythropoiesis and hepcidin suppression (91). This group of anemias includes some forms of hemolytic anemias such as dehydrated stomatocytosis 1 (DHS1) (92-94) and pyruvate kinase deficiency (PKD) (95,96), congenital dyserythropoietic anemias (CDAs; classified into the 3 major types-I, II, III and the transcription factor-related CDAs (97), congenital sideroblastic anemias like X-linked sideroblastic anemia (XLSA) (98), thalassemia and sickle cell disease (SCD) (Figure 3). These anemias are mostly normocytic ~ macrocytic, but in β-thalassemia minor it is microcytic. Also, in severe anemic cases, acquired transfusion-related iron overload occurs (13,78,79). One of the underestimated types of iron overload is DHS1 (92-94), which needs to be emphasized as a cause of non-transfusion-dependent iron overload. Iron overload in DSH1 (99-101) could be explained by chronic hemolysis, but the findings that PIEZO1 activation induced Ca2+ influx and suppression of HAMP expression causing low hepcidin indicate a link between PIEZO1 and iron metabolism (93). In these anemias, serum ferritin may be already high on non-transfusion status and become even higher after transfusions (102). In addition, extremely rare types of inherited microcytic anemias showing iron overload include congenital aceluroplasminemia (ACP), congenital atransferrinemia (ATF), and DMT1 deficiency (26,103-105) (Figure 3). ACP is characteristic of the brain, and other organ damages due to the accumulation of iron but with low serum Fe, normal TSAT, and microcytic anemia (103). ATF and DMT1 deficiency are characterized by iron-deficient erythropoiesis, severe microcytic anemia with high TSAT associated with decreased serum levels of transferrin and iron, and parenchymal iron overload due to secondary hepcidin suppression (26,104). Of these cases, ACP and ferroportin disease may belong to the diseases due to inefficient iron export from storage cells, while ATF and MDT1 deficiency to the diseases due to defects of iron delivery to maturing erythroblasts or erythroblast iron handling (26). These inherited microcytic anemias need to be differentiated from thalassemias and iron-refractory iron deficiency anemia (IRIDA) (106). Actual serum ferritin values were; from 815 to 5,141 ng/mL in DHS1 (99,101), median (ranges) of 228 (58–3,160) ng/mL in non-transfused patients with PKD (96), from 890 to 1,493 ng/mL in XLAS (98), 916±507 ng/mL in cases of post-transfusion CDA type I (107), 1,996 ng/mL in a case of CDA type II (108). If transfused cases were included; from 300 to >2,500 ng/mL in cases of SCD (109), and median (ranges) of 1,960 (44–21,828) ng/mL in the combined cases of SCD, thalassemia, and other congenital anemias (110). Serum ferritin values in ACP were; from 855 to 1,140 ng/mL (85,111), in ATF were; from 250 to 837 ng/mL (104), and from 58 to 305 ng/mL in DMT1 deficiency (105). Management policy for these disorders is similar to that in hemochromatosis.
Conclusions
From the clinical point of view, various conditions of hyperferritinemia with or without iron overload have been overviewed. Physicians most frequently encounter patients with hyperferritinemia due to infectious/inflammatory causes: Especially, HLH and MAS as the most severe forms in which markedly high serum ferritin (>3,000 ng/mL) is caused by rapid synthesis and release into sera under the cytokine effect. On the other hand, hemochromatosis and inherited iron-loading anemias represent hyperferritinemia associated with iron overload. In these diseases, serum ferritin is mild to moderately elevated and becomes significantly higher after transfusions. It is not infrequent that middle-aged adult patients with metabolic hyperferritinemia are referred to hematologists as unknown hyperferritinemia. Rare hereditary hyperferritinemia such as HHCS is known to be without iron overload and shows mild to moderate hyperferritinemia. Since mild to moderately elevated serum ferritin is most frequently noted with various conditions (Figure 2), careful differentiation is required from clinical information and with the use of serum Fe, TSAT, and imaging studies. From a future perspective, assays of hepcidin and glycosylated ferritin status should be available in daily practice. In addition, target gene sequencing analysis is encouraged to be applied broadly for unexplained hyperferritinemia, especially for inherited disorders using a gene panel as listed in Table 2.
Acknowledgments
The author thanks Ms. Yasuko Hashimoto, Kyoto Prefectural University of Medicine, for her assistance in searching for references.
Funding: None.
Footnote
Peer Review File: Available at https://jlpm.amegroups.org/article/view/10.21037/jlpm-23-78/prf
Conflicts of Interest: The author has completed the ICMJE uniform disclosure form (available at https://jlpm.amegroups.org/article/view/10.21037/jlpm-23-78/coif). S.I. serves as an unpaid editorial board member of Journal of Laboratory and Precision Medicine from September 2023 to August 2025. The author has no other conflicts of interest to declare.
Ethical Statement: The author is accountable for all aspects of the work in ensuring that questions related to the accuracy or integrity of any part of the work are appropriately investigated and resolved.
Open Access Statement: This is an Open Access article distributed in accordance with the Creative Commons Attribution-NonCommercial-NoDerivs 4.0 International License (CC BY-NC-ND 4.0), which permits the non-commercial replication and distribution of the article with the strict proviso that no changes or edits are made and the original work is properly cited (including links to both the formal publication through the relevant DOI and the license). See: https://creativecommons.org/licenses/by-nc-nd/4.0/.
References
- Anderson GJ, Frazer DM. Current understanding of iron homeostasis. Am J Clin Nutr 2017;106:1559S-66S. [Crossref] [PubMed]
- Gao G, Li J, Zhang Y, et al. Cellular Iron Metabolism and Regulation. Adv Exp Med Biol 2019;1173:21-32. [Crossref] [PubMed]
- Knovich MA, Storey JA, Coffman LG, et al. Ferritin for the clinician. Blood Rev 2009;23:95-104. [Crossref] [PubMed]
- Sandnes M, Ulvik RJ, Vorland M, et al. Hyperferritinemia-A Clinical Overview. J Clin Med 2021;10:2008. [Crossref] [PubMed]
- Ferritin Worwood M. Blood Rev 1990;4:259-69. [Crossref] [PubMed]
- Plays M, Müller S, Rodriguez R. Chemistry and biology of ferritin. Metallomics 2021;13:mfab021. [Crossref] [PubMed]
- Rogers JT, Bridges KR, Durmowicz GP, et al. Translational control during the acute phase response. Ferritin synthesis in response to interleukin-1. J Biol Chem 1990;265:14572-8. [Crossref] [PubMed]
- Kwak EL, Larochelle DA, Beaumont C, et al. Role for NF-kappa B in the regulation of ferritin H by tumor necrosis factor-alpha. J Biol Chem 1995;270:15285-93. [Crossref] [PubMed]
- Zandman-Goddard G, Shoenfeld Y. Hyperferritinemia in autoimmunity. Isr Med Assoc J 2008;10:83-4. [PubMed]
- Raynor A, Peoc'h K, Boutten A. Measurement of glycosylated ferritin with Concanavalin A: Assay design, optimization and validation. J Chromatogr B Analyt Technol Biomed Life Sci 2022;1194:123184. [Crossref] [PubMed]
- Guerber A, Garneret E, El Jammal T, et al. Evaluation of Glycosylated Ferritin in Adult-Onset Still's Disease and Differential Diagnoses. J Clin Med 2022;11:5012. [Crossref] [PubMed]
- Kell DB, Pretorius E. Serum ferritin is an important inflammatory disease marker, as it is mainly a leakage product from damaged cells. Metallomics 2014;6:748-73. [Crossref] [PubMed]
- Beaton MD, Adams PC. Treatment of hyperferritinemia. Ann Hepatol 2012;11:294-300. [Crossref] [PubMed]
- Birgegård G. The source of serum ferritin during infection. Studies with concanavalin A--Sepharose absorption. Clin Sci (Lond) 1980;59:385-7. [Crossref] [PubMed]
- Cohen LA, Gutierrez L, Weiss A, et al. Serum ferritin is derived primarily from macrophages through a nonclassical secretory pathway. Blood 2010;116:1574-84. [Crossref] [PubMed]
- Kernan KF, Carcillo JA. Hyperferritinemia and inflammation. Int Immunol 2017;29:401-9. [Crossref] [PubMed]
- Celma Nos F, Hernández G, Ferrer-Cortès X, et al. Hereditary Hyperferritinemia Cataract Syndrome: Ferritin L Gene and Physiopathology behind the Disease-Report of New Cases. Int J Mol Sci 2021;22:5451. [Crossref] [PubMed]
- Kannengiesser C, Jouanolle AM, Hetet G, et al. A new missense mutation in the L ferritin coding sequence associated with elevated levels of glycosylated ferritin in serum and absence of iron overload. Haematologica 2009;94:335-9. [Crossref] [PubMed]
- Monfrini E, Pelucchi S, Hollmén M, et al. A form of inherited hyperferritinemia associated with bi-allelic pathogenic variants of STAB1. Am J Hum Genet 2023;110:1436-43. [Crossref] [PubMed]
- Kowdley KV, Belt P, Wilson LA, et al. Serum ferritin is an independent predictor of histologic severity and advanced fibrosis in patients with nonalcoholic fatty liver disease. Hepatology 2012;55:77-85. [Crossref] [PubMed]
- Henter JI, Horne A, Aricó M, et al. HLH-2004: Diagnostic and therapeutic guidelines for hemophagocytic lymphohistiocytosis. Pediatr Blood Cancer 2007;48:124-31. [Crossref] [PubMed]
- Ravelli A, Minoia F, Davì S, et al. 2016 Classification Criteria for Macrophage Activation Syndrome Complicating Systemic Juvenile Idiopathic Arthritis: A European League Against Rheumatism/American College of Rheumatology/Paediatric Rheumatology International Trials Organisation Collaborative Initiative. Arthritis Rheumatol 2016;68:566-76. [Crossref] [PubMed]
- Hankins JS, McCarville MB, Loeffler RB, et al. R2* magnetic resonance imaging of the liver in patients with iron overload. Blood 2009;113:4853-5. [Crossref] [PubMed]
- Taher AT, Viprakasit V, Musallam KM, et al. Treating iron overload in patients with non-transfusion-dependent thalassemia. Am J Hematol 2013;88:409-15. [Crossref] [PubMed]
- Sieni E, Cetica V, Hackmann Y, et al. Familial hemophagocytic lymphohistiocytosis: when rare diseases shed light on immune system functioning. Front Immunol 2014;5:167. [Crossref] [PubMed]
- Piperno A, Pelucchi S, Mariani R. Inherited iron overload disorders. Transl Gastroenterol Hepatol 2020;5:25. [Crossref] [PubMed]
- Imashuku S. Differential diagnosis of hemophagocytic syndrome: underlying disorders and selection of the most effective treatment. Int J Hematol 1997;66:135-51. [Crossref] [PubMed]
- Davì S, Consolaro A, Guseinova D, et al. An international consensus survey of diagnostic criteria for macrophage activation syndrome in systemic juvenile idiopathic arthritis. J Rheumatol 2011;38:764-8. [Crossref] [PubMed]
- Aytaç S, Batu ED, Ünal Ş, et al. Macrophage activation syndrome in children with systemic juvenile idiopathic arthritis and systemic lupus erythematosus. Rheumatol Int 2016;36:1421-9. [Crossref] [PubMed]
- Goda K, Kenzaka T, Hoshijima M, et al. Adult-onset Still's disease with macrophage activation syndrome diagnosed and treated based on cytokine profiling: a case-based review. Rheumatol Int 2020;40:145-52. [Crossref] [PubMed]
- Thoufeeq MH, Ali Khan SL, Jain SK, et al. A case of acute infectious mononucleosis presenting with very high ferritin. World J Gastroenterol 2007;13:637-8. [Crossref] [PubMed]
- Theodory B, Dopp M, Swisher AR, et al. Epstein-Barr virus induced acute hepatitis with hyperferritinemia: A rare presentation. IDCases 2023;33:e01872. [Crossref] [PubMed]
- Dematapitiya C, Perera C, Chinthaka W, et al. Cold type autoimmune hemolytic anemia- a rare manifestation of infectious mononucleosis; serum ferritin as an important biomarker. BMC Infect Dis 2019;19:68. [Crossref] [PubMed]
- Cheng L, Li H, Li L, et al. Ferritin in the coronavirus disease 2019 (COVID-19): A systematic review and meta-analysis. J Clin Lab Anal 2020;34:e23618. [Crossref] [PubMed]
- Deng F, Zhang L, Lyu L, et al. Increased levels of ferritin on admission predicts intensive care unit mortality in patients with COVID-19. Med Clin (Engl Ed) 2021;156:324-31. [Crossref] [PubMed]
- Kaushal K, Kaur H, Sarma P, et al. Serum ferritin as a predictive biomarker in COVID-19. A systematic review, meta-analysis and meta-regression analysis. J Crit Care 2022;67:172-81. [Crossref] [PubMed]
- Fang YP, Zhang HJ, Guo Z, et al. Effect of Serum Ferritin on the Prognosis of Patients with Sepsis: Data from the MIMIC-IV Database. Emerg Med Int 2022;2022:2104755. [Crossref] [PubMed]
- He L, Guo C, Su Y, et al. The relationship between serum ferritin level and clinical outcomes in sepsis based on a large public database. Sci Rep 2023;13:8677. [Crossref] [PubMed]
- Parodi A, Davì S, Pringe AB, et al. Macrophage activation syndrome in juvenile systemic lupus erythematosus: a multinational multicenter study of thirty-eight patients. Arthritis Rheum 2009;60:3388-99. [Crossref] [PubMed]
- La Rosée F, Bremer HC, Gehrke I, et al. The Janus kinase 1/2 inhibitor ruxolitinib in COVID-19 with severe systemic hyperinflammation. Leukemia 2020;34:1805-15. [Crossref] [PubMed]
- Esumi N, Ikushima S, Todo S, et al. Hyperferritinemia in malignant histiocytosis and virus-associated hemophagocytic syndrome. N Engl J Med 1987;316:346-7. [Crossref] [PubMed]
- Rajantie J, Rapola J, Siimes MA. Ferritinemia with subnormal iron stores in lysinuric protein intolerance. Metabolism 1981;30:3-5. [Crossref] [PubMed]
- Esumi N, Ikushima S, Todo S, et al. Hyperferritinemia in malignant histiocytosis, virus-associated hemophagocytic syndrome and familial erythrophagocytic lymphohistiocytosis. A survey of pediatric cases. Acta Paediatr Scand 1989;78:268-70. [Crossref] [PubMed]
- Allen CE, Yu X, Kozinetz CA, et al. Highly elevated ferritin levels and the diagnosis of hemophagocytic lymphohistiocytosis. Pediatr Blood Cancer 2008;50:1227-35. [Crossref] [PubMed]
- Knaak C, Nyvlt P, Schuster FS, et al. Hemophagocytic lymphohistiocytosis in critically ill patients: diagnostic reliability of HLH-2004 criteria and HScore. Crit Care 2020;24:244. [Crossref] [PubMed]
- Kapoor S. Distinguishing hemophagocytic lymphohistiocytosis from hemochromatosis in patients with hyperferritinemia. Pediatr Blood Cancer 2008;50:1287-author reply 1287-8. [Crossref] [PubMed]
- Ishihara A, Yamauchi T, Ikeda K, et al. Glycosylated ferritin as an improved marker for post-transfusion iron overload. Int J Hematol 2021;113:537-46. [Crossref] [PubMed]
- Lambotte O, Cacoub P, Costedoat N, et al. High ferritin and low glycosylated ferritin may also be a marker of excessive macrophage activation. J Rheumatol 2003;30:1027-8. [PubMed]
- Wang Z, Wang Y, Wang J, et al. Early diagnostic value of low percentage of glycosylated ferritin in secondary hemophagocytic lymphohistiocytosis. Int J Hematol 2009;90:501-5. [Crossref] [PubMed]
- Nabergoj M, Marinova M, Binotto G, et al. Diagnostic and prognostic value of low percentage of glycosylated ferritin in acquired hemophagocytic lymphohistiocytosis: A single-center study. Int J Lab Hematol 2017;39:620-4. [Crossref] [PubMed]
- Bergsten E, Horne A, Aricó M, et al. Confirmed efficacy of etoposide and dexamethasone in HLH treatment: long-term results of the cooperative HLH-2004 study. Blood 2017;130:2728-38. [Crossref] [PubMed]
- Albeituni S, Verbist KC, Tedrick PE, et al. Mechanisms of action of ruxolitinib in murine models of hemophagocytic lymphohistiocytosis. Blood 2019;134:147-59. [Crossref] [PubMed]
- Goldsmith SR, Saif Ur Rehman S, Shirai CL, et al. Resolution of secondary hemophagocytic lymphohistiocytosis after treatment with the JAK1/2 inhibitor ruxolitinib. Blood Adv 2019;3:4131-5. [Crossref] [PubMed]
- Wang H, Gu J, Liang X, et al. Low dose ruxolitinib plus HLH-94 protocol: A potential choice for secondary HLH. Semin Hematol 2020;57:26-30. [Crossref] [PubMed]
- Locatelli F, Jordan MB, Allen C, et al. Emapalumab in Children with Primary Hemophagocytic Lymphohistiocytosis. N Engl J Med 2020;382:1811-22. [Crossref] [PubMed]
- Cheloff AZ, Al-Samkari H. Emapalumab for the treatment of hemophagocytic lymphohistiocytosis. Drugs Today (Barc) 2020;56:439-46. [Crossref] [PubMed]
- Gabr JB, Liu E, Mian S, et al. Successful treatment of secondary macrophage activation syndrome with emapalumab in a patient with newly diagnosed adult-onset Still's disease: case report and review of the literature. Ann Transl Med 2020;8:887. [Crossref] [PubMed]
- Garonzi C, Chinello M, Cesaro S. Emapalumab for adult and pediatric patients with hemophagocytic lymphohistiocytosis. Expert Rev Clin Pharmacol 2021;14:527-34. [Crossref] [PubMed]
- Horne A, Janka G, Maarten Egeler R, et al. Haematopoietic stem cell transplantation in haemophagocytic lymphohistiocytosis. Br J Haematol 2005;129:622-30. [Crossref] [PubMed]
- Cazzola M, Bergamaschi G, Tonon L, et al. Hereditary hyperferritinemia-cataract syndrome: relationship between phenotypes and specific mutations in the iron-responsive element of ferritin light-chain mRNA. Blood 1997;90:814-21. [Crossref] [PubMed]
- Luscieti S, Tolle G, Aranda J, et al. Novel mutations in the ferritin-L iron-responsive element that only mildly impair IRP binding cause hereditary hyperferritinaemia cataract syndrome. Orphanet J Rare Dis 2013;8:30. [Crossref] [PubMed]
- Levi S, Girelli D, Perrone F, et al. Analysis of ferritins in lymphoblastoid cell lines and in the lens of subjects with hereditary hyperferritinemia-cataract syndrome. Blood 1998;91:4180-7. [Crossref] [PubMed]
- Perruccio K, Arcioni F, Cerri C, et al. The hereditary hyperferritinemia-cataract syndrome in 2 italian families. Case Rep Pediatr 2013;2013:806034. [Crossref] [PubMed]
- Thurlow V, Vadher B, Bomford A, et al. Two novel mutations in the L ferritin coding sequence associated with benign hyperferritinaemia unmasked by glycosylated ferritin assay. Ann Clin Biochem 2012;49:302-5. [Crossref] [PubMed]
- Yang Y, Lin T, Kuang P, et al. Ferritin L-subunit gene mutation and hereditary hyperferritinaemia cataract syndrome (HHCS): a case report and literature review. Hematology 2021;26:896-903. [Crossref] [PubMed]
- Fargion S, Mattioli M, Fracanzani AL, et al. Hyperferritinemia, iron overload, and multiple metabolic alterations identify patients at risk for nonalcoholic steatohepatitis. Am J Gastroenterol 2001;96:2448-55. [Crossref] [PubMed]
- Valenti L, Fracanzani AL, Dongiovanni P, et al. Iron depletion by phlebotomy improves insulin resistance in patients with nonalcoholic fatty liver disease and hyperferritinemia: evidence from a case-control study. Am J Gastroenterol 2007;102:1251-8. [Crossref] [PubMed]
- Brudevold R, Hole T, Hammerstrøm J. Hyperferritinemia is associated with insulin resistance and fatty liver in patients without iron overload. PLoS One 2008;3:e3547. [Crossref] [PubMed]
- Datz C, Müller E, Aigner E. Iron overload and non-alcoholic fatty liver disease. Minerva Endocrinol 2017;42:173-83. [PubMed]
- Barisani D, Pelucchi S, Mariani R, et al. Hepcidin and iron-related gene expression in subjects with Dysmetabolic Hepatic Iron Overload. J Hepatol 2008;49:123-33. [Crossref] [PubMed]
- Deugnier Y, Bardou-Jacquet É, Lainé F. Dysmetabolic iron overload syndrome (DIOS). Presse Med 2017;46:e306-11. [Crossref] [PubMed]
- Rametta R, Fracanzani AL, Fargion S, et al. Dysmetabolic Hyperferritinemia and Dysmetabolic Iron Overload Syndrome (DIOS): Two Related Conditions or Different Entities? Curr Pharm Des 2020;26:1025-35. [Crossref] [PubMed]
- Sachinidis A, Doumas M, Imprialos K, et al. Dysmetabolic Iron Overload in Metabolic Syndrome. Curr Pharm Des 2020;26:1019-24. [Crossref] [PubMed]
- Makker J, Hanif A, Bajantri B, et al. Dysmetabolic hyperferritinemia: all iron overload is not hemochromatosis. Case Rep Gastroenterol 2015;9:7-14. [Crossref] [PubMed]
- Chen LY, Chang SD, Sreenivasan GM, et al. Dysmetabolic hyperferritinemia is associated with normal transferrin saturation, mild hepatic iron overload, and elevated hepcidin. Ann Hematol 2011;90:139-43. [Crossref] [PubMed]
- Rauber MR, Pilger DA, Cecconello DK, et al. Hepcidin is a useful biomarker to evaluate hyperferritinemia associated with metabolic syndrome. An Acad Bras Cienc 2019;91:e20180286. [Crossref] [PubMed]
- Valenti L, Corradini E, Adams LA, et al. Consensus Statement on the definition and classification of metabolic hyperferritinaemia. Nat Rev Endocrinol 2023;19:299-310. [Crossref] [PubMed]
- Pantopoulos K. Inherited Disorders of Iron Overload. Front Nutr 2018;5:103. [Crossref] [PubMed]
- Gattermann N. The treatment of secondary hemochromatosis. Dtsch Arztebl Int 2009;106:499-504. I. [PubMed]
- Olynyk JK, Ramm GA. Hemochromatosis. N Engl J Med 2022;387:2159-70. [Crossref] [PubMed]
- Adams PC, Jeffrey G, Ryan J. Haemochromatosis. Lancet 2023;401:1811-21. [Crossref] [PubMed]
- Ikuta K, Hatayama M, Addo L, et al. Iron overload patients with unknown etiology from national survey in Japan. Int J Hematol 2017;105:353-60. [Crossref] [PubMed]
- Adams PC, Reboussin DM, Barton JC, et al. Hemochromatosis and iron-overload screening in a racially diverse population. N Engl J Med 2005;352:1769-78. [Crossref] [PubMed]
- Lim A, Speechley M, Adams PC. Predicting C282Y homozygote genotype for hemochromatosis using serum ferritin and transferrin saturation values from 44,809 participants of the HEIRS study. Can J Gastroenterol Hepatol 2014;28:502-4. [Crossref] [PubMed]
- Hattori A, Miyajima H, Tomosugi N, et al. Clinicopathological study of Japanese patients with genetic iron overload syndromes. Pathol Int 2012;62:612-8. [Crossref] [PubMed]
- Griffiths WJH, Besser M, Bowden DJ, et al. Juvenile haemochromatosis. Lancet Child Adolesc Health 2021;5:524-30. [Crossref] [PubMed]
- Majore S, Bonaccorsi di Patti MC, Valiante M, et al. Characterization of three novel pathogenic SLC40A1 mutations and genotype/phenotype correlations in 7 Italian families with type 4 hereditary hemochromatosis. Biochim Biophys Acta Mol Basis Dis 2018;1864:464-70. [Crossref] [PubMed]
- Santiago de Sousa Azulay R, Magalhães M, Tavares MDG, et al. Novel Mutation in the Hemojuvelin Gene (HJV) in a Patient with Juvenile Hemochromatosis Presenting with Insulin-dependent Diabetes Mellitus, Secondary Hypothyroidism and Hypogonadism. Am J Case Rep 2020;21:e923108. [Crossref] [PubMed]
- Lescano MA, Tavares LC, Santos PCJL. Juvenile hemochromatosis: HAMP mutation and severe iron overload treated with phlebotomies and deferasirox. World J Clin Cases 2017;5:381-3. [Crossref] [PubMed]
- Palmer WC, Vishnu P, Sanchez W, et al. Diagnosis and Management of Genetic Iron Overload Disorders. J Gen Intern Med 2018;33:2230-6. [Crossref] [PubMed]
- Pagani A, Nai A, Silvestri L, et al. Hepcidin and Anemia: A Tight Relationship. Front Physiol 2019;10:1294. [Crossref] [PubMed]
- Zarychanski R, Schulz VP, Houston BL, et al. Mutations in the mechanotransduction protein PIEZO1 are associated with hereditary xerocytosis. Blood 2012;120:1908-15. [Crossref] [PubMed]
- Albuisson J, Murthy SE, Bandell M, et al. Dehydrated hereditary stomatocytosis linked to gain-of-function mutations in mechanically activated PIEZO1 ion channels. Nat Commun 2013;4:1884. [Crossref] [PubMed]
- Andolfo I, Rosato BE, Manna F, et al. Gain-of-function mutations in PIEZO1 directly impair hepatic iron metabolism via the inhibition of the BMP/SMADs pathway. Am J Hematol 2020;95:188-97. [Crossref] [PubMed]
- Bianchi P, Fermo E. Molecular heterogeneity of pyruvate kinase deficiency. Haematologica 2020;105:2218-28. [Crossref] [PubMed]
- Zanella A, Berzuini A, Colombo MB, et al. Iron status in red cell pyruvate kinase deficiency: study of Italian cases. Br J Haematol 1993;83:485-90. [Crossref] [PubMed]
- Iolascon A, Andolfo I, Russo R. Congenital dyserythropoietic anemias. Blood 2020;136:1274-83. [Crossref] [PubMed]
- Lira Zidanes A, Marchi G, Busti F, et al. A Novel ALAS2 Missense Mutation in Two Brothers With Iron Overload and Associated Alterations in Serum Hepcidin/Erythroferrone Levels. Front Physiol 2020;11:581386. [Crossref] [PubMed]
- Imashuku S, Muramatsu H, Sugihara T, et al. PIEZO1 gene mutation in a Japanese family with hereditary high phosphatidylcholine hemolytic anemia and hemochromatosis-induced diabetes mellitus. Int J Hematol 2016;104:125-9. [Crossref] [PubMed]
- Orvain C, Da Costa L, Van Wijk R, et al. Inherited or acquired modifiers of iron status may dramatically affect the phenotype in dehydrated hereditary stomatocytosis. Eur J Haematol 2018;101:566-9. [Crossref] [PubMed]
- Imashuku S, Suemori SI, Wakamatsu M, et al. Juvenile Hemochromatosis With Non-transfused Hemolytic Anemia Caused by a De Novo PIEZO1 Gene Mutation. J Pediatr Hematol Oncol 2023;45:e510-3. [Crossref] [PubMed]
- Yassin M, Soliman A, De Sanctis V, et al. Liver Iron Content (LIC) in Adults with Sickle Cell Disease (SCD): Correlation with Serum Ferritin and Liver Enzymes Concentrations in Trasfusion Dependent (TD-SCD) and Non-Transfusion Dependent (NT-SCD) Patients. Mediterr J Hematol Infect Dis 2017;9:e2017037. [Crossref] [PubMed]
- Vila Cuenca M, Marchi G, Barqué A, et al. Genetic and Clinical Heterogeneity in Thirteen New Cases with Aceruloplasminemia. Atypical Anemia as a Clue for an Early Diagnosis. Int J Mol Sci 2020;21:2374. [Crossref] [PubMed]
- Shamsian BS, Rezaei N, Arzanian MT, et al. Severe hypochromic microcytic anemia in a patient with congenital atransferrinemia. Pediatr Hematol Oncol 2009;26:356-62. [Crossref] [PubMed]
- Mims MP, Guan Y, Pospisilova D, et al. Identification of a human mutation of DMT1 in a patient with microcytic anemia and iron overload. Blood 2005;105:1337-42. [Crossref] [PubMed]
- Cappellini MD, Russo R, Andolfo I, et al. Inherited microcytic anemias. Hematology Am Soc Hematol Educ Program 2020;2020:465-70. [Crossref] [PubMed]
- Tamary H, Shalev H, Perez-Avraham G, et al. Elevated growth differentiation factor 15 expression in patients with congenital dyserythropoietic anemia type I. Blood 2008;112:5241-4. [Crossref] [PubMed]
- Tamura H, Matsumoto G, Itakura Y, et al. A case of congenital dyserythropoietic anemia type II associated with hemochromatosis. Intern Med 1992;31:380-4. [Crossref] [PubMed]
- Coates TD, Wood JC. How we manage iron overload in sickle cell patients. Br J Haematol 2017;177:703-16. [Crossref] [PubMed]
- Lobo C, Angulo IL, Aparicio LR, et al. Retrospective epidemiological study of Latin American patients with transfusional hemosiderosis: the first Latin American epidemiological study in iron overload--the RELATH study. Hematology 2011;16:265-73. [Crossref] [PubMed]
- Kaneko Y, Miyajima H, Piperno A, et al. Measurement of serum hepcidin-25 levels as a potential test for diagnosing hemochromatosis and related disorders. J Gastroenterol 2010;45:1163-71. [Crossref] [PubMed]
Cite this article as: Imashuku S. Clinical evaluation of hyperferritinemia with or without iron overload. J Lab Precis Med 2024;9:16.