Use of iron deficiency flag of the hematology analyzer Sysmex XN-3000 for early iron-deficient erythropoiesis diagnosis
Highlight box
Key findings
• The results of our investigation suggest that flag Iron-Deficiency (Iron-Def) may be used as an easy, inexpensive and rapid index for initial screening for iron-deficient erythropoiesis.
What is known and what is new?
• At the present, this flag Iron-Def is automatically calculated by the XN haematological analyser but is not included in the iron-deficient erythropoiesis diagnostic criteria.
• Our results, even if only preliminary, suggest that flag Iron-Def may be used as a rapid index for initial screening for iron-deficient erythropoiesis.
What is the implication, and what should change now?
• This flag Iron-Def could be used as an initial screening for an early iron-deficient erythropoiesis diagnosis. However, further studies are needed to confirm these preliminary findings.
Introduction
Iron deficiency (ID) is currently regarded as a major global healthcare issue, given that approximately 2 billion people (i.e., over 30% of the worldwide population) have been diagnosed with iron deficiency anaemia (IDA) (1). Several studies have shown that IDA remains prevalent in both developing and developed countries. This condition may be associated with poorer cognitive and neurophysiologic maturation in early life, developmental delays, behaviour and mental disorders, and perinatal complications in pregnant and premenopausal women (2).
Erythropoiesis involves the close interaction of iron and erythropoietin. In essence, erythropoietin is the accelerator that drives erythropoiesis. Iron is the fuel for the production of new red blood cells. When the two are coupled, red cell production moves briskly and efficiently. If one component is absent (e.g., ID) anemia results.
ID can be conventionally defined as a condition in which iron availability is insufficient to meet the body needs and can manifest with or without anaemia (2). Thus, ID is clearly underpinning why rapidly growing children and premenopausal women are at higher risk. Dietary insufficiency as a cause of ID is secondary to intestinal blood loss due to parasitizes in many countries around the world (3).
Early detection and prompt treatment of ID are both essential because of the crucial role played by iron in human physiology. Many studies have shown that ID is associated with longer stays in hospital, cognitive dysfunction, increased risk of falls, as well as with overall reduced life expectancy (4,5). In the presence of an adequate nutritional iron supply and without concomitant inflammatory conditions, systemic homeostasis maintains plasma iron levels between 10–30 µmol/L and whole-body iron stores between 0.3–1.0 g (6). The leading mechanism of iron homeostasis encompasses an interplay between hepcidin, a hormone produced by liver, and its receptor ferroportin, the iron exporter that allows the passage of iron from intestinal cells, macrophages and hepatocytes into the plasma (6).
The gold standard for ID diagnosis is the staining of bone marrow macrophages and erythroid precursor with Prussian blue in marrow aspiration. However, this procedure is quite expensive, invasive and subjective (7-10). Traditionally, serum ferritin, iron, transferrin, transferrin saturation and soluble transferrin receptor are used to diagnose ID. Nevertheless, these markers are affected by inflammatory processes and influenced by dietary intake and circadian variation (7-12). Due to these diagnostics difficulties, in 2005 the reticulocyte haemoglobin equivalent (RET-He) was introduced. However, in most laboratory medicine services, the evaluation of this biomarker is rarely available even as an urgent test and is not performed by default on all patients. This leads to a delay in diagnosis of days with the various consequences mentioned previously. Therefore, the signalling of this flag could be useful in the rapid addition of RET-He.
The XN-3000 haematology analyzer automatically generates, in all samples analysed for complete blood count cells (CBC), a flag defined Iron-Deficiency (Iron-Def). This flag is generated by the red blood cell distribution width-coefficient of variation (RDW-CV), mean cell volume (MCV) and mean cell haemoglobin concentration (MCHC).
Therefore, the aim of our investigation was to evaluate the diagnostic performance of the Iron-Def flag and its possible role in the identification of patients with iron-deficient erythropoiesis. We present this article in accordance with the STARD reporting checklist (available at https://jlpm.amegroups.org/article/view/10.21037/jlpm-23-93/rc).
Methods
Study design
Hematological data of all samples for which CBC and reticulocytes were requested at the same time during a 12-month period (1st June 2022–30th June 2023) at the Clinical Pathology Laboratory, ASST Nord Milano, Milan, Italy, were retrospective extracted from the IPU (information processing unit) Sysmex database.
In addition to the extraction of the hematological parameters, the Iron-Def flag data were also extracted to evaluate its diagnostics performance in iron-deficient erythropoiesis identification. The Iron-Def flag parameter is always calculated by the system were a blood count is performed. This flag is calculated by using the RDW-CV, the MCHC and the MCV and values. The definitive underlying conditions of the flags are defined as “confidential” by Sysmex. This combination of parameters typically characterises microcytic hypochromic anaemia due to iron-deficient erythropoiesis. The presence of this flag should prompt additional investigations and confirmatory tests for the final diagnosis of iron-deficient erythropoiesis. No exclusion criteria were applied for this retrospective study.
Patients with RET-He values ≤25.7 pg were considered positive. This cut-off of ≤25.7 pg was acquired from work by Uçar and collaborators (13).
Blood samples
All samples were obtained from referred for blood testing. Blood samples were collected in dipotassium (k2) ethylenediaminetetraacetic acid (EDTA) tubes (Greiner Bio-One VacuetteTM, Kremsmünster, Austria). Hematological testing was performed immediately upon arrival in the laboratory, specifically, within 3 hours of collection for outpatients and within one hour for inpatients.
Analyser
The CBC was tested using a Sysmex XN-3000 haematology analyser (Sysmex, Kobe, Japan), which enumerates and classifies blood cells by means of flow cytometry. More specifically, cell enumeration is accomplished with semiconductor laser counting and cells are classified by irradiation with a 633 nm laser beam and analysis of forward scattered light, side scattered light and side fluorescent light. The three signals are integrated to differentiate and count. The performance of the Sysmex XN-series has been extensively evaluated by Seo et al., as described elsewhere (14,15).
Statistical analysis
The correlation among the flag Iron-Def and haematological parameters was analysed by the Spearman’s rank order test. Receiver operating characteristic (ROC) curves were constructed to evaluate the diagnostic performance of the Iron-Def value for detecting iron-deficient erythropoiesis. Statistical analysis was executed using the Analyze-it software (version 3.90.1).
Ethical statement
The study was conducted in accordance with the Declaration of Helsinki (as revised in 2013). This study is under the terms of all relevant local legislations. The data obtained from our study did not invalidate the patient’s clinic. Given the retrospective data analysis nature, informed consent was not required. Test results age and sex of patients were anonymously extracted from the database of the IPU. Formal approval of the protocol by the local Ethics Committee was considered unnecessary because the study did not interfere with the usual clinical routine.
Results
This study included 3,489 patients, the mean age of the study population was 46.6 years (standard deviation: ±25.9 years) and 60% of patients were men. Patient characteristics are summarized in Table 1.
Table 1
Variables | Values |
---|---|
Number of patients | 3,489 |
Men, n (%) | 2,094 (60.0) |
Women, n (%) | 1,395 (40.0) |
Age (years), mean ± SD | 46.6±25.9 |
RET-HE normal values, n | 2,891 |
†RET-HE abnormal values, n | 598 |
†, RET-HE ≤25.7 pg. SD, standard deviation; RET-HE, reticulocyte hemoglobin equivalent.
The Shapiro-Wilk test for normal distribution showed that all these parameters were non-normally distributed, so that they were displayed as median, 95% confidence interval (95% CI) and interquartile range (IQR). The value of the different parameters measured in this study shown in Table 2.
Table 2
Parameters | Shapiro-Wilk test for normal distribution | Median | 95% CI for the median | IQR |
---|---|---|---|---|
HGB (g/L) | Reject normality (P<0.005) | 112 | 111 to 113 | 29 |
MCHC (g/L) | Reject normality (P<0.001) | 332 | 323 to 333 | 23 |
MCH (pg) | Reject normality (P<0.001) | 29.6 | 29.5 to 29.8 | 5.3 |
MCV (fL) | Reject normality (P<0.001) | 89 | 88.4 to 89.5 | 15 |
MicroR% | Reject normality (P<0.001) | 2.8 | 2.7 to 3.0 | 6.6 |
MacroR% | Reject normality (P<0.001) | 4.0 | 3.9 to 4.0 | 2.2 |
RBC (×1012/L) | Reject normality (P<0.001) | 3.93 | 3.89 to 3.97 | 1.22 |
RDW-CV (%) | Reject normality (P<0.001) | 14.9 | 14.8 to 15.6 | 3.30 |
Flag (Iron-Def) | Reject normality (P<0.001) | 80 | 80 to 80 | 10 |
RET-HE | Reject normality (P<0.001) | 31.2 | 31.0 to 31.3 | 6 |
CI, confidence interval; IQR, interquartile range; HGB, haemoglobin; MCHC, mean cell haemoglobin concentration; MCH, mean cell haemoglobin; MCV, mean cell volume; MicroR%, percentage of microcytic red cells; MacroR%, percentage of macrocytic red cells; RBC, red blood cells; RDW, red blood cell distribution width; CV, coefficient of variation; Iron-Def, Iron-Deficiency; RET-HE, reticulocyte hemoglobin equivalent.
A significant negative correlation was observed between Iron-Def and haematocrit (HCT), haemoglobin (HGB), MCV, mean cell haemoglobin (MCH), percentage of macrocytic red cells (MacroR%), RET-He, whereas a positive correlation could be found with red blood cells (RBC), RDW-CV, percentage of microcytic red cells (MicroR%) (Table 3).
Table 3
Parameters | Rho | 95% CI | P value |
---|---|---|---|
RBC vs. flag (Iron-Def) | 0.302 | 0.268 to 0.335 | <0.001 |
HGB vs. flag (Iron-Def) | −0.246 | −0.280 to −0.211 | <0.001 |
HCT vs. flag (Iron-Def) | −0.190 | −0.226 to −0.155 | <0.001 |
MCV vs. flag (Iron-Def) | −0.869 | −0.877 to −0.859 | <0.001 |
MCH vs. flag (Iron-Def) | −0.837 | −0.848 to −0.826 | <0.001 |
MCHC vs. flag (Iron-Def) | −0.262 | −0.296 to −0.227 | <0.001 |
RDW-CV vs. flag (Iron-Def) | 0.150 | 0.114 to 0.186 | <0.001 |
MicroR% vs. flag (Iron-Def) | 0.792 | 0.778 to 0.805 | <0.001 |
MacroR% vs. flag (Iron-Def) | −0.676 | −0.696 to −0.656 | <0.001 |
RET-He vs. flag (Iron-Def) | −0.628 | −0.650 to −0.605 | <0.001 |
Iron-Def, Iron-Deficiency; Rho, Spearman’s coefficient of rank correlation; CI, confidence interval; RBC, red blood cells; HGB, haemoglobin; HCT, haematocrit; MCV, mean cell volume; MCH, mean cell haemoglobin; MCHC, mean cell haemoglobin concentration; RDW, red blood cell distribution width; CV, coefficient of variation; MicroR%, percentage of microcytic red cells; MacroR%, percentage of macrocytic red cells; RET-He, reticulocyte hemoglobin equivalent.
The evaluation of the diagnostic performance of flag Iron-Def for detecting RET-He values above and below 25.7 pg, due the ROC curve analysis, shows that the most accurate flag Iron-Def cut-off value was 80. This value showed a good diagnostic performance with the area under the curve (AUC) of 0.855 (95% CI: 0.837–0.873). Furthermore, the flag Iron-Def cut-off value 80 is characterized by an excellent specificity and sensibility of 0.737 and 0.891 respectively (Figure 1 and Table 4).
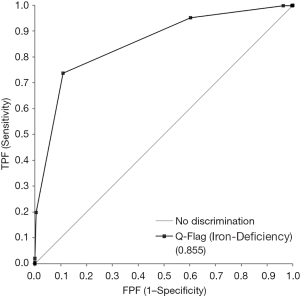
Table 4
Flag (Iron-Def) | Sens | Spec | TP | FP | TN | FN |
---|---|---|---|---|---|---|
20 | 1.000 | 0.000 | 598 | 2,890 | 1 | 0 |
30 | 0.998 | 0.001 | 597 | 2,887 | 4 | 1 |
40 | 0.998 | 0.002 | 597 | 2,885 | 6 | 1 |
50 | 0.998 | 0.004 | 597 | 2,880 | 11 | 1 |
60 | 0.998 | 0.037 | 597 | 2,784 | 107 | 1 |
70 | 0.952 | 0.397 | 569 | 1,742 | 1,149 | 29 |
80 | 0.737 | 0.891 | 441 | 315 | 2,576 | 157 |
90 | 0.197 | 0.997 | 118 | 10 | 2,881 | 480 |
100 | 0.020 | 1.000 | 12 | 0 | 2,891 | 586 |
110 | 0.005 | 1.000 | 3 | 0 | 2,891 | 595 |
120 | 0.002 | 1.000 | 1 | 0 | 2,891 | 597 |
130 | 0.000 | 1.000 | 0 | 0 | 2,891 | 598 |
Iron-Def, Iron-Deficiency; RET-He, reticulocyte hemoglobin equivalent; Sens, sensitivity; Spec, specificity; TP, true positive; FP, false positive; TN, true negative; FN, false negative.
Discussion
Despite the numerous diagnostic progress in laboratory haematology, the diagnosis of IDA still relies heavily on a limited number of traditional biomarkers (i.e., serum iron, ferritin, haemoglobin, transferrin). However, these biomarkers are affected by inflammatory processes, circadian variation and dietary intake (7).
Different scientific papers reported that RET-He is a marker providing a snapshot of iron availability for erythropoiesis in the bone marrow; it is an indicator of iron therapy response and an early marker of iron-deficient erythropoiesis (11,15,16). RET-He enables an early detection and indication of ID because of its shorter life span (1–2 days) in the circulation than mature erythrocytes (120 days), but also because of its better predictive value for ID if compared to hemocytometric parameters such as MCV, RDW and HGB (7,16-21). These last markers are insensitive in the detection of early erythropoietic disturbances as their changes (MCV: 21 days, RDW: 30 days, and HGB: 60 days) are observed when IDA has already taken place. In contrast, RET-He is the first peripheral blood count marker being abnormal in the presence of ID. It seems also to be an early indicator of iron therapy response, because its variations could be detected about 2 days after the initiation of the optimal treatments and in contrast to ferritin, for which the first response can be observed in 1–2 weeks (16,22-25)..
In another paper, Uçar et al., reported that RET-He (best cut-off ≤25.7 pg) is quite a useful marker in the diagnosis of ID and IDA, and in the assessment of treatment response, as well as in the differential diagnosis of ID and IDA since it gives results very quickly and is not influenced by other infectious and chronic conditions (13).
Unfortunately, RET-He is not currently performed on all outpatients and inpatients jet, and it is not considered an emergency test. However, the use of Iron-Def, such innovative diagnostic tools would be welcomed to obtain an earlier and more efficient identification of subjects at risk of this condition.
Muñoz et al. recently highlighted the importance of identifying patients with IDA in the emergency department (26). Based on a cost-analysis, treating patients with ID by administering ferric carboxymaltose may be a cost-saving approach compared with conventional outpatient care (27).
This study has explored the diagnostic performance of Iron-Def flag to identify patients with ID and IDA. Notably, this flag is automatically calculated by the XN haematological analyser, so that it could be provided without expenses along with other parameters of the CBC.
The results of our investigation shows that the most accurate flag Iron-Def cut-off value was 80. This cut-off showed a good diagnostic performance with the AUC of 0.855 (95% CI: 0.837–0.873) (Figure 1).
However, using a lower cut-off of the flag Iron-Def (i.e., 70) could be useful for screening patients with iron deficiencies. This cut-off of 70 showed a high sensitivity and a low specificity, respectively of 0.952 and 0.397 (showed in Table 4). The flag Iron-Def is clearly meant to be used as a screening tool, for which a high sensitivity is an excellent premise. The lower specificity implies that other test, such as RET-He should be used for a definitive diagnosis, within an integrated diagnostic approach in which Iron-Def may be placed at an initial stage (28,29).
A limitation of this study is the lack of complete clinical information on sample population, that could influence the calculated Iron-Def flag and unfortunately, we have no data to predict the behaviour of Iron-Def in other causes of hypochromic hypochromia, such as thalassaemia and other diseases. Furthermore, an important limitation is that no validation of these preliminary results was performed in a prospective manner.
Conclusions
In conclusion, the results of our investigation suggest that Iron-Def may be used as an easy, inexpensive and rapid index for initial screening for iron-deficient erythropoiesis. Further studies are needed to confirm these preliminary findings.
Acknowledgments
Funding: None.
Footnote
Reporting Checklist: The authors have completed the STARD reporting checklist. Available at https://jlpm.amegroups.org/article/view/10.21037/jlpm-23-93/rc
Data Sharing Statement: Available at https://jlpm.amegroups.org/article/view/10.21037/jlpm-23-93/dss
Peer Review File: Available at https://jlpm.amegroups.org/article/view/10.21037/jlpm-23-93/prf
Conflicts of Interest: All authors have completed the ICMJE uniform disclosure form (available at https://jlpm.amegroups.org/article/view/10.21037/jlpm-23-93/coif). The authors have no conflicts of interest to declare.
Ethical Statement: The authors are accountable for all aspects of the work in ensuring that questions related to the accuracy or integrity of any part of the work are appropriately investigated and resolved. The study was conducted in accordance with the Declaration of Helsinki (as revised in 2013). This study is under the terms of all relevant local legislations. The data obtained from our study did not invalidate the patient’s clinic. Given the retrospective data analysis nature, informed consent was not required. Test results age and sex of patients were anonymously extracted from the database of the IPU. Formal approval of the protocol by the local Ethics Committee was considered unnecessary because the study did not interfere with the usual clinical routine.
Open Access Statement: This is an Open Access article distributed in accordance with the Creative Commons Attribution-NonCommercial-NoDerivs 4.0 International License (CC BY-NC-ND 4.0), which permits the non-commercial replication and distribution of the article with the strict proviso that no changes or edits are made and the original work is properly cited (including links to both the formal publication through the relevant DOI and the license). See: https://creativecommons.org/licenses/by-nc-nd/4.0/.
References
- Cacoub P, Vandewalle C, Peoc'h K. Using transferrin saturation as a diagnostic criterion for iron deficiency: A systematic review. Crit Rev Clin Lab Sci 2019;56:526-32. [Crossref] [PubMed]
- Camaschella C. Iron-deficiency anemia. N Engl J Med 2015;372:1832-43. [Crossref] [PubMed]
- Cappellini MD, Comin-Colet J, de Francisco A, et al. Iron deficiency across chronic inflammatory conditions: International expert opinion on definition, diagnosis, and management. Am J Hematol 2017;92:1068-78. [Crossref] [PubMed]
- Andrews NC. Disorders of iron metabolism. N Engl J Med 1999;341:1986-95. [Crossref] [PubMed]
- Keshav S, Stevens R. New concepts in iron deficiency anaemia. Br J Gen Pract 2017;67:10-1. [Crossref] [PubMed]
- Ganz T. Anemia of Inflammation. N Engl J Med 2019;381:1148-57. [Crossref] [PubMed]
- Poffenroth C, Mabbett C, Kendrick C. The reticulocyte haemoglobin equivalent (RET_He) and laboratory screening for iron deficiency. New Zealand Journal of Medical Laboratory Science 2017;71:120-3.
- Mehta S, Goyal LK, Kaushik D, et al. Reticulocyte Hemoglobin vis-a-vis Serum Ferritin as a Marker of Bone Marrow Iron Store in Iron Deficiency Anemia. J Assoc Physicians India 2016;64:38-42. [PubMed]
- Braga F, Infusino I, Dolci A, et al. Soluble transferrin receptor in complicated anemia. Clin Chim Acta 2014;431:143-7. [Crossref] [PubMed]
- Lopez A, Cacoub P, Macdougall IC, et al. Iron deficiency anaemia. Lancet 2016;387:907-16. [Crossref] [PubMed]
- Dalimunthe NN, Lubis AR. Usefulness of Reticulocyte Hemoglobin Equivalent in Management of Regular Hemodialysis Patients with Iron Deficiency Anemia. Rom J Intern Med 2016;54:31-6. [Crossref] [PubMed]
- Löfving A, Domellöf M, Hellström-Westas L, et al. Reference intervals for reticulocyte hemoglobin content in healthy infants. Pediatr Res 2018;84:657-61. [Crossref] [PubMed]
- Uçar MA, Falay M, Dağdas S, et al. The Importance of RET-He in the Diagnosis of Iron Deficiency and Iron Deficiency Anemia and the Evaluation of Response to Oral Iron Therapy. J Med Biochem 2019;38:496-502. [Crossref] [PubMed]
- Daves M, Zagler EM, Cemin R, et al. Sample stability for complete blood cell count using the Sysmex XN haematological analyser. Blood Transfus 2015;13:576-82. [PubMed]
- Seo JY, Lee ST, Kim SH. Performance evaluation of the new hematology analyzer Sysmex XN-series. Int J Lab Hematol 2015;37:155-64. [Crossref] [PubMed]
- Buttarello M. Laboratory diagnosis of anemia: are the old and new red cell parameters useful in classification and treatment, how? Int J Lab Hematol 2016;38:123-32. [Crossref] [PubMed]
- Schoorl M. Innovative haematological parameters in clinical practice. Ned Tijdschr Klin Chem Labgeneesk 2016;41:6-16.
- Sunkara A, Kotta DR. Evaluation of Red Cell Indices and Reticulocyte Maturity Indices Including Reticulocyte Haemoglobin Concentration in Iron Deficiency Anaemia in Adult Female Population. Journal of Evidence Based Medicine and Healthcare 2016;3:5315-8. [Crossref]
- Levy S, Schapkaitz E. The clinical utility of new reticulocyte and erythrocyte parameters on the Sysmex XN 9000 for iron deficiency in pregnant patients. Int J Lab Hematol 2018;40:683-90. [Crossref] [PubMed]
- Joosten E, Lioen P, Brusselmans C, et al. Is analysis of the reticulocyte haemoglobin equivalent a useful test for the diagnosis of iron deficiency anaemia in geriatric patients? Eur J Intern Med 2013;24:63-6. [Crossref] [PubMed]
- Abdul Gafor AH, Subramaniam R, Hadi F, et al. The Role of Reticulocyte Hemoglobin Content in the Management of Iron Deficiency Anemia in Patients on Hemodialysis. Nephro-Urol Mon 2018;10:e65629. [Crossref]
- Wollmann M, Gerzson BM, Schwert V, et al. Reticulocyte maturity indices in iron deficiency anemia. Rev Bras Hematol Hemoter 2014;36:25-8. [Crossref] [PubMed]
- Doig K. Disorders of iron kinetics and heme metabolism. In: Keohane EM, Jm W, Smith LJ. editors. Rodak’s Hematology: Clinical Principles and Applications (5th edition). St. Louis, MO, USA: Elsevier Saunders; 2016:297-305.
- Schoorl M, Schoorl M, van der Gaag D, et al. Effects of iron supplementation on red blood cell hemoglobin content in pregnancy. Hematol Rep 2012;4:e24. [Crossref] [PubMed]
- Powers JM, Buchanan GR. Diagnosis and management of iron deficiency anemia. Hematol Oncol Clin North Am 2014;28:729-45. vi-vii. [Crossref] [PubMed]
- Muñoz M, Gómez-Ramírez S, Besser M, et al. Current misconceptions in diagnosis and management of iron deficiency. Blood Transfus 2017;15:422-37. [PubMed]
- Quintana-Díaz M, Muñoz-Romo R, Gómez-Ramírez S, et al. A fast-track anaemia clinic in the Emergency Department: cost-analysis of intravenous iron administration for treating iron-deficiency anaemia. Blood Transfus 2017;15:438-46. [PubMed]
- Urrechaga E, Boveda O, Aguayo FJ, et al. Percentage of hypochromic erythrocytes and reticulocyte hemoglobin equivalent predictors of response to intravenous iron in hemodialysis patients. Int J Lab Hematol 2016;38:360-5. [Crossref] [PubMed]
- Roccaforte V, Daves M, Lippi G, et al. Preliminary assessment of the new Sysmex XN parameter Iron-Def for identifying iron deficiency. Blood Transfus 2020;18:406-12. [PubMed]
Cite this article as: Roccaforte V, Lombardi F, Panella R, Salamone A, Daves M, De Martino A, Spreafico M, Piccin A, Pastori S. Use of iron deficiency flag of the hematology analyzer Sysmex XN-3000 for early iron-deficient erythropoiesis diagnosis. J Lab Precis Med 2024;9:19.