Investigative algorithms for disorders causing hypercalcaemia and hypocalcaemia: a narrative review
Introduction
Calcium is the fifth most abundant element (1) and the most abundant electrolyte (2) in the human body. Calcium is essential for normal physiological functioning, including blood clotting, bone mineralisation, muscle contraction, hormone secretion, and nerve conductivity (3). Identifying calcium abnormalities is essential to minimise potentially significant morbidity and mortality associated with hypercalcaemia and hypocalcaemia. However, accurately assessing calcium status can be difficult for reasons that are discussed. Laboratory focused diagnostic algorithms are presented to help healthcare professionals identify principle causes of hypercalcaemia and hypocalcaemia. These algorithms will not discuss treatment and are not designed to replace local guidelines or specialist endocrinological knowledge/advice. Instead, these algorithms are most useful as general diagnostic aids when faced with a patient who has a calcium derangement of unknown cause.
A detailed review of calcium balance and homeostasis is beyond the scope of this review; please see existing comprehensive reviews for more information (1-5). However, a simplified summary is presented here to provide context for the clinical interpretation of laboratory results. We present this article in accordance with the Narrative Review reporting checklist (available at https://jlpm.amegroups.org/article/view/10.21037/jlpm-24-6/rc).
Methods
The narrative literature review was created by searching Medline, PubMed, Google Scholar and OMIM. The diagnostic algorithms were then created using the information gathered from the literature review as well as expert opinion of the authors. Literature was searched over the period August 2023 to December 2023 and language was restricted to English. For further information, please see Table 1.
Table 1
Items | Specification |
---|---|
Date of search | August 2023 to December 2023 |
Databases and other sources searched | Medline, PubMed, Google Scholar and OMIM |
Search terms used | Calcium, hypercalcaemia, hypocalcaemia, drugs, investigations, algorithms, guidelines, diagnosis, causes, aetiology, human, physiology, hyperparathyroidism, total calcium, ionised calcium, blood gas analyser, homeostasis, parathyroid hormone, vitamin D, toxicity, deficiency |
Timeframe | August 2023 to December 2023 |
Inclusion criteria | Restricted to English |
Selection process | K.C.F. and K.E.S. conducted initial search, with refinement by all other authors to obtain consensus and agreement |
Any additional considerations, if applicable | Seminal texts were also searched and the references of important articles and texts were obtained and checked for relevance |
Background
Calcium balance
The majority of healthy adults are in a net zero calcium balance due to neutral bone turnover as demonstrated in Figure 1 (2,5), therefore dietary consumption and excretion are approximately equal. However, net calcium balance is heavily influenced by the longer-term rates of bone turnover. For example, children are usually in a net positive (less excretion than consumption) calcium balance due to greater bone formation in order to facilitate growth (1). Conversely, older people are often in a net negative (less consumption than excretion) calcium balance due to the loss of bone mass associated with ageing (1). It is important to note that Figure 1 is a simplification of a complicated process (6), and calcium balance becomes particularly more complex in patients with chronic kidney disease (CKD) (7).
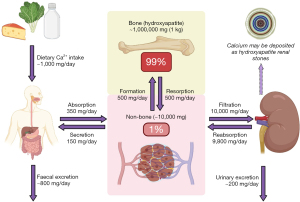
Importantly, the only source of calcium is from the diet (1). Dietary requirements range from approximately 500 to 1,500 mg/day depending on age (1,8), and individuals ≥60 years of age may require supplements to meet daily calcium intake demands (9) due to poorer absorption from the diet and declining hormonal homeostatic regulation (3). However, recent studies have demonstrated that sufficient calcium intake in order to minimise the risk of fractures can be achieved purely by consuming food sufficient in calcium (700 mg/day) and protein without the need for pharmacological supplementation (10).
Calcium distribution
Greater than 99% of the approximate 1 kg of total calcium in the body is located in the skeleton, mostly in the form of hydroxyapatite (calcium phosphate complexes), see Figure 2 (1). Consequently, any process that influences the rate of bone formation or reabsorption can quickly lead to hypercalcaemia or hypocalcaemia. Of the remaining 1% (~10 g) of total body calcium, 99% (0.99% of total body calcium) is located intracellularly, and 1% (0.01% of total body calcium) is located extracellularly (11). The extracellular calcium is further divided into the circulating blood fraction—which is usually maintained at a concentration between 2.2–2.6 mmol/L (8.8–10.4 mg/dL)—and the interstitial fluid fraction (1).
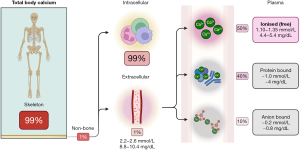
Blood calcium fractions
Of the total calcium located in the blood, approximately 50% is ionised (free), 40% is bound to proteins (80% albumin and 20% globulins) (11), and 10% is bound to anions including phosphate, oxalate, citrate, lactate and carbonate, see Figure 2 (1,3,12). It is the blood ionised (free) calcium that is the ‘biologically active’ form as it is immediately available to enter cells to exert its physiological effects (3). This is opposed to blood protein and anion bound calcium, which are considered ‘biologically inactive’. Homeostatic mechanisms act to tightly maintain blood ionised calcium between 1.10–1.35 mmol/L (4.4–5.4 mg/dL); this constitutes approximately half of the blood total calcium concentration (1).
The appropriateness of the general use of the term ‘ionised’ calcium has been debated for decades because all calcium—whether it is free or bound to proteins/anions—is technically chemically ionised (Ca2+) (13). Consequently, it is more appropriate to refer to the classical ‘ionised’ calcium as ‘free’ or ‘unbound’ calcium. Despite this, to avoid confusion and for consistency with the literature, free calcium will be referred to as ionised calcium throughout this review.
Blood calcium methods
Most calcium methods utilised in central laboratories measure the total calcium (serum or plasma), which includes all three forms of calcium (ionised, protein bound and anion bound). However, as previously discussed, it is usually only the ionised calcium fraction that is clinically relevant, therefore total calcium concentrations are often not a true reflection of calcium homeostasis. This is particularly apparent in the presence of any factors that affect the concentration of total protein in the blood, particularly albumin (e.g., lower in inflammation, nephrotic syndrome, malnutrition etc.) (14), as the total calcium will shift in the same direction with less (or even no) associated change in ionised calcium.
Additionally, clinically important changes in blood ionised calcium can occur even when there is no net gain or loss of blood total calcium (resulting in no change in the laboratory total calcium result), which occurs during acidaemia and alkalaemia due to the buffering properties of protein (mainly albumin), see Figure 3 (15). During acidaemia, there is more competition from free hydrogen ions (H+) for binding sites on blood proteins (15), decreasing ionised calcium binding and therefore more free calcium to exert biological effect. For every 0.1 unit decrease in blood pH, blood ionised calcium increases between 0.036–0.050 mmol/L (0.14–0.20 mg/dL) (15,16). Conversely, during alkalaemia, there is less competition from H+ for binding sites on blood proteins, increasing ionised calcium binding and therefore less free calcium.
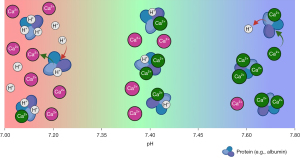
Blood ionised calcium can be directly measured using ion selective electrodes found in blood gas analysers (17), and this is a more accurate reflection of the clinically important calcium status than total plasma calcium methods (18). However, routine ionised calcium measurement is notoriously difficult as the concentrations are rapidly and significantly affected by pH (as described above), patient posture, recent dietary intake, circadian rhythm, temperature variation etc. (17). Therefore, strict sample requirements—including blood collection into heparinised tubes, and analysis within 30 minutes or capping the sample to avoid CO2 loss and the resulting pH changes—must be met for reliable results (17). Consequently, although a poorer reflection of true calcium status, laboratory analysis of total calcium remains the most common method of calcium analysis due to much easier routine measurement and reduced cost (17).
Laboratory adjusted calcium
To reduce the effect of variable biologically inactive protein bound calcium on laboratory total calcium result interpretation, most laboratories ‘adjust’ the total calcium result to the albumin concentration using population specific equations. Although the term ‘corrected’ calcium is still in widespread use throughout the literature, this term should be avoided as it may give a false sense that albumin-adjusted total calcium results are ‘correct’. However, it is well documented that this is frequently not the case due to the assumptions that are made when adjusting. A full discussion of the limitations of albumin-adjusted calcium is beyond the scope of this review and has already been covered extensively by Jassam et al. in their recent series in this journal (19-23). However, for context, important limitations include (but are not limited to) lack of adjustment equation standardisation (20), significant variation based on bromocresol green and bromocresol purple albumin methods (24), poor calcium adjustment performance at very low and very high concentrations of albumin, and poor performance in critically and acutely sick patients (25). It has therefore been rightfully proposed that direct ionised calcium analysis should ideally replace total calcium analysis (26). Argument for the abandonment of any adjustment of total calcium results in favour of ionised calcium analysis instead (when total calcium is abnormal) has even been put forward (27). However, as albumin-adjustment of total calcium is still common, this will be included in the following algorithms.
Calcium homeostasis
As mentioned above, homeostatic mechanisms actively work to maintain a tight ionised (free) calcium within the reference interval (1.10–1.35 mmol/L) (1). The two major hormones regulating calcium homeostasis are parathyroid hormone (PTH) and the activated form of vitamin D, 1,25-dihydroxyvitamin D, see Figure 4 (1). Importantly, the secretion of PTH from parathyroid glands in response to blood ionised calcium is a negative feedback mechanism controlled via the calcium sensing receptor (CaSR) (1). A fall in blood ionised calcium inactivates the CaSRs, leading to the secretion of PTH (28). PTH has three main actions via the parathyroid hormone receptor (PTHR), all of which increase blood ionised calcium:
- Stimulates bone-resorbing osteoclasts to release stored calcium and phosphate into the blood (28);
- Alters permeability of the renal distal tubule, leading to increased calcium and decreased phosphate reabsorption from the filtrate (28);
- Upregulates renal 1α-hydroxylase, leading to increased conversion of inactive 25-hydroxyvitamin D to active 1,25-dihydroxyvitamin D (29).
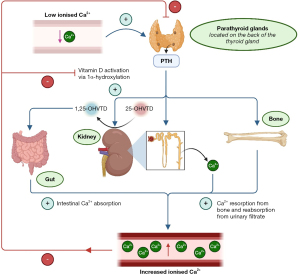
As the concentration of 1,25-dihydroxyvitamin D increases in response to a fall in blood ionised calcium, it ‘classically’ acts in conjunction with PTH to further increase blood ionised calcium by acting on vitamin D receptors (VDRs) in multiple tissues. ‘Classical’ actions of 1,25-dihydroxyvitamin D include:
- Increasing calcium and phosphate absorption in the intestines [by stimulating the expression of transient receptor potential cation channel subfamily V member 6 (TRPV6), and type II sodium-dependent phosphate cotransporter (Npt2b), respectively] (28);
- Increasing calcium reabsorption from the renal filtrate in the distal nephron (28);
- Acting on osteoblasts in bone to increase receptor activator of nuclear factor kappa beta ligand (RANKL) expression, which in turn interacts with RANK on osteoclasts to activate them and increase bone (and therefore calcium) resorption (28).
When blood ionised calcium increases because of the above actions of PTH and 1,25-dihydroxyvitamin D, the whole homeostatic mechanism is inhibited via increased stimulation of CaSRs leading to decreased PTH secretion (1). Additionally, 1,25-dihydroxyvitamin D also directly inhibits the secretion of PTH (28).
The hormone, calcitonin, also plays a role in calcium homeostasis. Calcitonin is released from the thyroid parafollicular C cells in response to an increase in blood ionised calcium and acts to decrease bone and tubular calcium reabsorption (30) to prevent hypercalcaemia. Despite this, calcitonin absence after thyroidectomy does not lead to hypercalcaemia alone (30), therefore measurement of calcitonin in the diagnostic work up of calcium abnormalities has no established use in current routine practice.
Hypercalcaemia
Hypercalcaemia is a common finding in clinical practice, with an annual incidence of 0.6% in hospitalised patients (31) and an approximate prevalence of 0.1% in the general population (32). It develops when calcium entry into the plasma exceeds the rate of bone deposition and renal excretion, outlined in Figure 1 (33). Biochemical categorisation of hypercalcaemia based on blood calcium concentration is shown in Table 2 (33), but the utility of this in clinical practice is limited. Factors such as rate of increase, hydration status, age, and comorbidities will affect the severity of symptoms (34).
Table 2
Blood calcium fraction | Mild | Moderate | Severe |
---|---|---|---|
Albumin-adjusted total calcium, mmol/L (mg/dL) | 2.61–2.99 (10.5–11.9) | 3.00–3.49 (12.0–13.9) | ≥3.50 (≥14.0) |
Ionised calcium, mmol/L (mg/dL) | 1.36–1.99 (5.5–7.9) | 2.00–2.49 (8.0–9.9) | ≥2.50 (≥10.0) |
Consequently, clinicians must consider the overall clinical presentation in conjunction with the blood results, including how quickly the calcium concentration has changed at lower concentrations, when determining the urgency of investigation and treatment. Generally, mild hypercalcaemia is frequently asymptomatic or is associated with mild constipation or fatigue, and is usually due to primary hyperparathyroidism (PHPT) (33). Moderate hypercalcaemia can also present mildly if it is chronic but, similarly to severe hypercalcaemia and/or sudden increases at lower ionised calcium concentrations, it is more likely to present with the above as well as polyuria, polydipsia, dehydration, altered mental state, muscle weakness and abnormal electrocardiogram (ECG) [prolonged PR interval, short QT interval, widened QRS complex, arrhythmia] (33). More severe presentations and higher concentrations of ionised calcium are more likely to be due to malignancy, or other causes, rather than PHPT.
Ninety percent of hypercalcaemia presentations are caused by either PHPT or malignancy (35), with PHPT making up >50% of all cases (16). See Table 3 for a summary of the differences between typical clinical presentations of PHPT and malignancy. Although not as commonly encountered, the remaining 10% of other causes are still clinically important to identify and address, and perhaps present healthcare professionals with the greatest difficulty in diagnosis. See Figure 5 for a proposed algorithm for the laboratory investigation of hypercalcaemia. However, it is essential to note that it is likely appropriate to skip the first couple of confirmatory steps if the clinical presentation and/or severely high calcium result increases the urgency of the situation, or the clinical history/presentation increases confidence that the initial laboratory hypercalcaemic assessment is genuine.
Table 3
Clinical presentation | Primary hyperparathyroidism | Malignancy |
---|---|---|
Serum adjusted calcium | Usually <3.0 mmol/L | May be ≥3.5 mmol/L |
Duration of hypercalcaemia | Long term | Acute/sub-acute |
Patient status | Relatively well | Acutely unwell |
Evidence of underlying malignancy | No | Often detected in a known malignancy, but can be the first clinical presentation if severe |
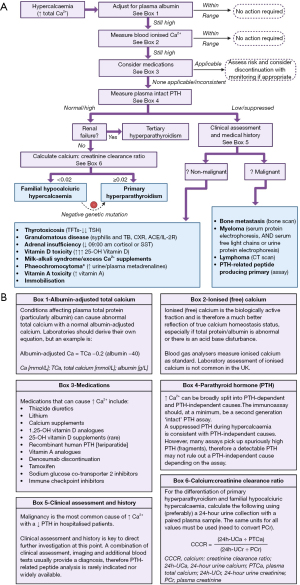
Confirming hypercalcaemia
A high total calcium may not be reflective of a high ionised calcium in the presence of disorders affecting total protein and albumin. A common equation that laboratories use to adjust total calcium—often called the ‘Payne’ equation—is shown in Figure 5B (box 1). Albumin adjustment can be done manually using this equation, or a more appropriate locally derived one, if adjustment is not done as part of the local laboratory service.
As the ionised calcium is the clinically important fraction, it can be useful to measure the ionised calcium in cases where the albumin-adjusted calcium is less reliable and/or the albumin-adjusted calcium does not fit with the clinical picture. This can be done using a blood gas analyser. Although central laboratory analysis of ionised calcium is not common in the United Kingdom (UK) due to analytical and cost limitations, it may be more commonly available in other countries. If the ionised calcium is discrepant to the albumin-adjusted total calcium results, it may be due to unrelated acute sickness, low albumin, etc. (14).
Medications that can cause hypercalcaemia
For a list of medications that can elevate calcium, see Figure 5B (box 3). It may be appropriate to stop potentially causal medications, review the dose, or monitor depending on the severity of hypercalcaemia, requirement of the medication and availability of alternatives. Although vitamin A supplements are traditionally associated with hypercalcaemia, it is very rarely seen (36). The mechanisms of drug-induced hypercalcaemia have been covered by Walker (33) and Lecoq (36).
PTH analysis
Plasma PTH analysis is a critically informative additional blood investigation in hypercalcaemia. If the PTH is inappropriately normal or high during hypercalcaemia, the diagnosis is likely PHPT or familial hypocalciuric hypercalcaemia (FHH) as the high ionised calcium should quickly decrease PTH secretion (Figure 4). If the PTH is appropriately low or suppressed, this suggests that a non-PTH mediated process is causing an increase in blood calcium, meaning that the diagnosis is much more likely to be malignancy or one of the less common causes outlined in Figure 5.
Biologically active PTH consists of amino acids 1 to 84 (37), and antibodies utilised in immunoassays can be directed at different amino acid fractions within this sequence. Second generation ‘intact’ PTH immunoassays utilise two polyclonal antibodies [one against the C-terminal (against amino acids 39–84) and one against the N-terminal (against amino acids 12–24 or 26–32 depending on the assay)] (37). Although an improvement over first generation immunoassays, the N-terminal antibody can still cross react with biologically inactive fragments consisting of amino acids 5 to 84. Despite this, second generation tests are widely used and are broadly sufficient (37). Third generation immunoassays—often called ‘whole’ or ‘bio-intact’ PTH assays—have very similar C-terminal antibodies to second generation assays but the N-terminal antibody only reacts with amino acids 1 to 4 (37). Therefore, third generation immunoassays only react with biologically active bio-intact PTH with the full 1 to 84 sequence of amino acids.
PTH dependent hypercalcaemia
If PTH is inappropriately elevated during hypercalcaemia, the likely diagnosis is PHPT, 80% of which are caused by a benign adenoma of one parathyroid gland (30). However, the possibility of FHH (a rare benign autosomal dominant mutation of CASR causing inactivation of CaSR) should not be disregarded as this does not require parathyroid surgical intervention (38). Current data suggest that 10% to 23% of unsuccessful parathyroidectomies can be attributed to FHH (38). There should be a higher index of suspicion for FHH if the patient is <40 years old, there is a family history of hypercalcaemia (33), the hypercalcaemia has always been present and static, and/or the calcium:creatinine clearance ratio (CCCR) is <0.01 (38,39). Importantly, urine calcium is highly variable and so the performance of the CCCR formula can be unreliable; neither biochemical markers nor genetics have perfect sensitivity or specificity (39).
During hypercalcaemia, FHH is traditionally associated with a PTH concentration within the reference interval, a CCCR of <0.01 and normo- or hypermagnesaemia (38,39), whereas PHPT is traditionally associated with an elevated PTH, a CCCR ≥0.01 and hypomagnesaemia. Importantly however, approximately 35% of genetically confirmed FHH cases have a CCCR of ≥0.01, 24% have elevated PTH, and the overlap of magnesium concentration is large between the two diagnoses (38,39). Additionally, 4% of PHPT patients may be misdiagnosed with FHH using a CCCR cut off of <0.01 (38). Testing for significant CASR mutations could be completed when the CCCR is <0.02 instead, which may identify up to 98% of FHH cases (38). Variants of unknown significance are common, and FHH with negative significant CASR mutation is always possible, therefore it may be appropriate to investigate strong family history more closely (39). The equation to calculate CCCR is shown in Figure 5B (box 5) (38).
The impact of CKD and chronic kidney disease-mineral and bone disorder (CKD-MBD) on calcium homeostasis and bone metabolism are important to understand (40). A full description of renal bone disease is outside the scope of this article. However, for context, due to the loss of renal function in CKD (with inability to activate vitamin D), PTH secretion increases in order to maintain normocalcaemia (secondary hyperparathyroidism). The increased PTH secretion can become autonomous, eventually leading to tertiary hyperparathyroidism if not well treated. This is a particular issue after a kidney transplant, where the sudden return of vitamin D activation capacity, in addition to the continued autonomous secretion of PTH, can cause rapid and significant hypercalcaemia. Tertiary hyperparathyroidism should be suspected by a background of CKD, with very high PTH concentrations and hypercalcaemia (40,41).
PTH independent hypercalcaemia
If PTH is appropriately low or suppressed (depending on the degree of hypercalcaemia), then the cause is most likely malignancy, particularly in hospitalised patients and if the hypercalcaemia is acute and/or severe (35). A thorough medical assessment and clinical history is particularly essential at this point to determine if the cause is more likely to be malignant or non-malignant (some of which are outlined in Figure 5).
Malignant hypercalcaemia with low/suppressed PTH
Approximately 20% of all cases of malignancy will present with hypercalcaemia at some point throughout their course (32) via one of the following mechanisms in order of frequency (30):
- PTH related protein (PTHrp) production by tumour (~80% of malignant causes);
- Bone metastasis (mainly from breast cancer or myeloma, ~20% of malignant causes);
- Expression of 1α-hydroxylase leading to production of excess 1,25-dihydroxyvitamin D (can occur in lymphoma);
- Ectopic PTH production (very rare, but can occur in small cell lung cancer, causing an inappropriately normal or high PTH instead of an appropriately low PTH) (42).
PTHrp, produced rarely by many carcinomas, is structurally similar to PTH and has many of the same physiological effects (42). However, PTHrp does not have significant effect on the production of 1,25-dihydroxyvitamin D (43). Although a low/suppressed PTH with an elevated PTHrp is consistent with a malignant cause of hypercalcaemia, PTHrp analysis is rarely indicated as the primary malignant cause is usually clinically evident (33). PTHrp analysis is very difficult and is not widely available, nor is it detected by routine PTH assays.
PTH-independent hypercalcaemia with complaints of bone pain (particularly in the lower back or sternum), and/or concurrent renal dysfunction and/or anaemia, should initiate investigation for myeloma (44). The greater the patient’s age, the greater the pre-screening likelihood of myeloma as the median age of diagnosis is 66–70 years old, and there is a frequency of only 0.02% to 0.3% in those younger than 30 years old (45). The laboratory screening tests should include serum protein electrophoresis and serum free light chains or urine protein electrophoresis to identify any present paraproteins (44). See the international myeloma working group 2014 guidelines for more information (44)
Malignant bone metastasis from other malignancies should also be considered and investigated by bone scan where appropriate. However, it is also important to appreciate that concurrent PHPT is responsible for 6% to 21% of cases of hypercalcemia among patients with cancer (46). A detailed review of the diagnostic work up of people with suspected malignancy include Mirrakhimov (42).
Non-malignant hypercalcaemia with low/suppressed PTH
Thyrotoxicosis should be considered as a rare cause of hypercalcaemia, therefore measurement of serum thyroid function tests (TFTs) may be required. TFTs traditionally include thyroid stimulating hormone (TSH) (also called thyrotropin) and free thyroxine (FT4). However, it is now common for TFTs to include TSH only as a first line test, which would be low/suppressed if thyrotoxicosis is present (47). If there is concurrent hypotension with hyponatraemia (and possible hyperkalaemia), adrenal insufficiency should be ruled out by a sufficiently high serum cortisol at 09:00 am (see local cut-offs), or by a short Synacthen® test as gold standard if clinical suspicion is high or the screening morning cortisol result is concerningly low (48,49).
Vitamin D toxicity from very high and prolonged supplementation of 25-OH vitamin D can rarely occur when plasma concentrations typically exceed 375 nmol/L (150 ng/mL) (50). The concentration of 1,25-OH vitamin D in the serum can be variable in toxicity (50,51), therefore its measurement is usually not helpful. Exogenous preparations of 1α-hydroxylated vitamin D preparations increase the risk of toxicity by bypassing hydroxylation regulation (50). However, hypercalcaemia from vitamin D supplementation is exceedingly rare. In one study, only 0.1% of patients taking 25-OH vitamin D supplements had a concentration >300 nmol/L (120 ng/mL), and only 4% of these (0.005% of all tested patients) had symptoms of vitamin D toxicity (51). Due to this rarity, confirmation of toxicity via measurement of vitamin D is very rarely required and the diagnosis should be clinically lead.
Granulomatous disease (including sarcoidosis and tuberculosis) can cause hypercalcaemia (and more commonly hypercalciuria) via increased autonomous production of 1,25-dihydroxyvitamin D (high hydroxylase activity in the granuloma) (52). There is no single test to conclusively diagnose sarcoidosis, therefore diagnosis is based on the histological presence of granulomas and exclusion of other causes of granulomatous inflammation (53), although IL-2 receptor is being increasingly used (54). Elevated serum angiotensin converting enzyme (ACE) is not a useful criterion for diagnosis as the sensitivity is only approximately 40% and it has false positive rate of 15% (53). However, ACE can be a useful monitoring marker, but more useful blood markers have been proposed, and many laboratories no longer offer ACE testing (55). Tuberculosis infection is the main differential for granulomatous disease, and depending on clinical history (56,57), testing is required and commonly available (58). Another re-emerging differential is syphilis, which can also present with granulomas, and tests are easily available in most laboratories.
Milk-alkali syndrome (MAS) (also called calcium-alkali syndrome) is a triad of hypercalcaemia, metabolic alkalosis and acute kidney injury (AKI) (59). MAS should be considered in patients taking high doses of calcium supplements (usually as a remedy for gastritis), particularly calcium carbonate which provides an absorbable alkali (59). A diagnosis of MAS should prompt cessation of calcium containing supplementation and alternative gastritis treatment.
Phaeochromocytoma is a very rare cause of hypercalcaemia, and the patient may have episodic or persistent hypertension. Phaeochromocytoma is most commonly a benign adenoma of adrenal chromaffin cells causing autonomous secretion of catecholamines, and is often linked to hereditary multiple endocrine neoplasia (MEN) syndrome (60) or other genetic susceptibility. Phaeochromocytoma can also be sporadically malignant (61). Plasma metadrenaline (also called metanephrine) analysis has slightly improved sensitivity and specificity than 24-hour urine metadrenaline excretion analysis for aiding the diagnosis of phaeochromocytoma and paraganglioma (60). However, 24-hour urine analysis is easier to complete in outpatient and primary care settings (62), so is usually the first test of choice in this context.
Although a very rare endocrinological route of presentation of hypercalcaemia (36), vitamin A toxicity should be considered where more common causes have been excluded, particularly if the patient is known to be taking vitamin A supplements and has CKD (63,64). The possibility of vitamin A toxicity is often evident by clinical history (including the consumption of retinoid drugs, mostly used in dermatology practice, e.g., bexarotene, acitretin and alitretinoin). If blood of vitamin A is required, plasma samples must be centrifuged quickly, and the plasma should be protected from light before analysis (65).
Extended periods (and sudden episodes in young males with peak bone mass) of immobilisation can also significantly increase bone turn-over, potentially causing extreme hypercalcaemia (66). However, this is rare and should be a diagnosis of exclusion in an appropriate clinical context, where increased concentrations of bone turnover blood markers, such as procollagen type 1 N-terminal propeptide (P1NP) and C-terminal telopeptide (CTX), may support a diagnosis (66).
Hypocalcaemia
Hypocalcaemia is rare in the general population, but has a prevalence of 15% in hospitalised patients and 85% in patients in intensive care units (67). The most common cause of hypocalcaemia in a primary care ambulant population (where hypocalcaemia is rare) is vitamin D deficiency, which in turn may have a prevalence as high as 50% in some populations (67). If hypocalcaemia develops slowly, it is frequently asymptomatic. However, acute development and/or severe cases (albumin-adjusted calcium <1.90 mmol/L or ionised calcium <0.95 mmol/L) can present with muscle cramps, seizures, arrhythmias and prolongation of the QT interval on ECG (68).
See Figure 6 for a proposed algorithm for the laboratory investigation of hypocalcaemia. However, the first couple of confirmatory steps (e.g., measurement of ionised calcium) may not be required if the low total calcium result is obviously genuine.
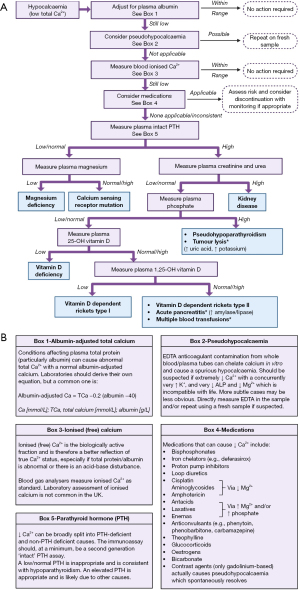
Confirming hypocalcaemia
A low blood total calcium should be confirmed by adjusting for albumin concentration. If still low, further confirmation by measuring ionised calcium may be warranted.
Pseudohypocalcaemia
If the low albumin-adjusted total calcium result is particularly unexpected and does not fit with the clinical presentation, then blood sample contamination from ethylenediaminetetraacetic acid (EDTA)—a common anticoagulant used in blood tubes for full blood count (also called complete blood count)—should be considered. EDTA often contains potassium and works as an anticoagulant by chelating calcium, but it also chelates magnesium and the cofactors required for alkaline phosphatase (ALP) activity. Consequently, a concurrent low magnesium, low ALP activity and elevated potassium (69) should increase suspicion of EDTA contamination, especially if extreme. More subtle contamination can be missed however (70), therefore a fresh sample should be obtained if in doubt.
Contamination of blood samples with the contents of oxalate and citrate containing blood tubes can also cause falsely low calcium results. It is therefore prudent to adhere to recommended order of draw and venesection protocols to minimise the risk of contamination. Citrate is also used in blood units as an anticoagulant.
Medications that can cause hypocalcaemia
Review patients’ current medications. Medications shown in Figure 6B (box 4) are causally linked to hypocalcaemia. It may be appropriate to stop such medication, review the dose or monitor, depending on the severity of hypocalcaemia, requirement of the medication and availability of alternatives. The mechanism of how these drugs cause hypocalcaemia has been covered by Liamis et al. (71).
PTH analysis
Like hypercalcaemia, plasma PTH analysis is the most informative additional blood investigation that can be performed during hypocalcaemia. PTH should normally rise quickly in the presence of hypocalcaemia to increase blood ionised calcium (Figure 4). If PTH is inappropriately low or normal, causes of hypoparathyroidism should be investigated. If PTH is appropriately elevated, other causes—outlined in Figure 6—should be considered.
Hypoparathyroidism
Hypoparathyroidism is usually caused by damage to the parathyroid glands, but abnormal parathyroid gland development or direct causes of reduced PTH secretion/activity (72) may also be seen. The cause of hypoparathyroidism may be evident from the clinical history. Thyroid and parathyroid surgery are a common cause (72). Autoimmune hypoparathyroidism is the next most common cause, which can be present in patients with adrenal insufficiency or Hashimoto thyroiditis (73). In some cases, anti-parathyroid gland and/or anti-CaSR antibody measurement in an expert centre may be helpful (73), but reported sensitivity and specificity is variable. Genetic causes of isolated hypoparathyroidism can be inherited in recessive, dominant or X-linked mechanisms, and can also be present in syndromic conditions such as DiGeorge syndrome (72). See Hassan-Smith et al. (72) and Bilezikian et al. (73) for more information.
Magnesium works as a negative regulator of PTH similar to calcium, where mild magnesium deficiency increases PTH secretion (74). However, severe magnesium deficiency (usually <0.5 mmol/L) paradoxically blocks PTH secretion and can result in severe hypocalcaemia (74). Hypocalcaemia is often refractory to treatment in cases of concurrent hypomagnesaemia, therefore magnesium normalisation is required before normocalcaemia can be maintained (67). Although hypomagnesaemia has been reported in up to 65% of intensive care patients (67), genuine total body magnesium deficiency is difficult to identify due to the unreliability of total serum magnesium assays in acutely sick patients (75). Hypomagnesaemia is only likely to be a cause of hypocalcaemia in patients with long term existing risk factors for deficiency including proton pump inhibitor use, gastrointestinal disease, poor intake, alcoholism, etc. (75).
Although very rare, CaSR mutations should be considered if hypoparathyroidism is present and is not due to the above (67); for more detail, see Bilezikian et al. (73).
Renal function assessment
If PTH is appropriately elevated, blood creatinine (with or without blood urea) should be measured and the estimated glomerular filtration rate (eGFR) calculated. If abnormal, this likely points towards renal dysfunction, which can cause hypocalcaemia due to reduced renal 1α-hydroxylase activity and therefore decreased synthesis of 1,25-dihydroxyvitamin D. Additionally, the increased plasma phosphate in renal dysfunction can cause calcium phosphate deposition in soft tissue (68).
Plasma phosphate
If renal function is not abnormal, plasma phosphate analysis may be a helpful next step. Phosphate is usually low when PTH activity is appropriately high (e.g., in secondary hyperparathyroidism as a result of vitamin D deficiency or osteomalacia), or high when PTH activity is inappropriately low (68). Pseudohypoparathyroidism is a rare end organ resistance to the effects of PTH, and can cause a very similar biochemical pattern to hypoparathyroidism except for a high plasma PTH (76). Pseudohypoparathyroidism should be suspected if PTH and phosphate are elevated during hypocalcaemia, and hypomagnesaemia, vitamin D deficiency and renal dysfunction have been ruled out, particularly in young patients (76). If suspected, GNAS mutation analysis may be helpful (G protein coupled to PTH receptor), but this is not always required for a diagnosis (76), see Linglart et al. (76) for more information.
If the patient has known malignancy that has rapidly progressed, or treatment has been recently given (particularly in leukaemia and lymphoma), tumour lysis syndrome (TLS) should be considered. TLS occurs when intracellular contents of tumour cells are rapidly released into circulation, causing elevated phosphate (which causes hypocalcaemia due to formation of calcium phosphate salts), potassium and uric acid (77).
Plasma 25-OH vitamin D analysis
As a major function of vitamin D is to stimulate intestinal calcium and phosphate absorption, vitamin D deficiency is a potential cause of hypocalcaemia. Despite this, 25-OH vitamin D testing in acutely sick patients should be discouraged as concentrations are directly related to vitamin D binding protein which is a negative acute phase reactant (78). Consequently, 25-OH vitamin D concentrations can be spuriously low in acutely sick patients, and may lead to unnecessary replacement (79,80) and diagnostic confusion. Analysis of 25-OH vitamin D can be a potential marker of metabolic bone disease (e.g., osteomalacia, rickets), especially if also considering risk factors for deficiency including low sunlight exposure, deeply pigmented skin, poor dietary intake, poor absorption etc.
Although 25-OH vitamin D concentrations <25 nmol/L (10 ng/mL) and <50 nmol/L (20 ng/mL) are frequently quoted as deficient and insufficient respectively, using strict cut-offs to determine when calcium absorption is directly compromised is unreliable. This is because routine immunoassays used to measure 25-OH vitamin D can produce significantly different results due to varying degrees of cross reactivity with metabolites and different forms (81). Consequently, the need for concurrent calcium replacement should be a clinical decision based on risk factors for both calcium and vitamin D deficiency, as well as the presence of symptoms of deficiency, rather than purely as a result of the vitamin D concentration in isolation.
Plasma 1,25-dihydroxyvitamin D analysis
Plasma 1,25-dihydroxyvitamin D analysis is rarely required; renal dysfunction can be inferred from other tests—e.g., creatinine—and the test is difficult to perform and is not widely available. Its role is limited to diagnosis of rare congenital conditions such as 1α-hydroxylase deficiency and vitamin D-dependent rickets type 1 (an autosomal recessive disorder). The presentation of such diagnoses is in childhood through bone deformities and hypocalcaemia; these cases should be referred to specialist centres for investigation and management. Decreased dietary consumption of calcium results in a homeostatic increase in plasma 1,25-dihydroxyvitamin D in attempt to increase intestinal absorption, and vice versa (82).
Acute pancreatitis and blood transfusions
The exact mechanism of hypocalcaemia as a result of pancreatitis is unknown, and evidence suggests that the mechanism differs between the early and late stage of the disease (83). If pancreatitis is a suspected cause of hypocalcaemia, plasma amylase or plasma lipase are helpful blood tests for further investigation. Although either test is usually acceptable to rule out pancreatitis in severe hypocalcaemia, lipase is generally the preferred marker due to its longer diagnostic window and improved sensitivity and specificity (84).
Severe hypocalcaemia can result after massive blood transfusion due to chelation from the anticoagulant citrate contained in the blood product units (85). Blood transfusion as a cause of hypocalcaemia is almost always obvious from the clinical history and is therefore unlikely to cause diagnostic confusion.
Special states
Pregnancy causes an apparent decrease in total calcium concentrations due to the haemodilution of albumin. Normally, the ionised calcium concentration should not change (86). The developing fetal skeleton requires calcium, therefore pregnant women have a net positive calcium balance (partly since the placenta secretes PTHrp) and a newborn baby typically has between 20–30 g of calcium (87). Calcium supplementation during pregnancy reduces risks of pre-eclampsia in those who have poor intake and improves maternal outcomes (88).
The algorithms (Figures 5,6) above suit most cases, however neonatal presentations may require a different approach. For example, rare inborn errors of metabolism may present, including Jansen metaphyseal chondrodysplasia—caused by an activating mutation of the PTH receptor—Williams syndrome, idiopathic infantile hypercalcaemia and hypophosphatasia (89). Hypophosphataemia in neonates can cause hypercalcaemia (90), as can subcutaneous fat necrosis (91). Maternal factors can influence neonatal metabolism, e.g., vitamin D toxicity or deficiency. Referral to specialist endocrinological centres should occur if these rare causes of calcium abnormalities are suspected.
Conclusions
The investigation of hypercalcaemia and hypocalcaemia can be challenging. As well as multiple factors that can cause results that are ‘fake’ or ‘spurious’ and not representative of the true in vivo calcium status, many conditions can cause variable calcium concentrations depending on a host of factors. Here, diagnostic algorithms are presented that should help the reader to systematically consider causes of calcium disturbances in a logical order, with perhaps albumin-adjusted calcium followed by PTH analysis being the most important informative steps. These algorithms cannot replace specialist knowledge, experience and local guidelines, and rarer causes should be referred to specialist centres. Instead, they should act as a diagnostic aid when assessing patients with calcium disturbances.
Acknowledgments
We would like to thank Professor Rousseau Gama for inspiring and inviting us to prepare this paper.
Funding: None.
Footnote
Provenance and Peer Review: This article was commissioned by the editorial office, Journal of Laboratory and Precision Medicine for the series “Investigative Algorithms in Laboratory Medicine II: Focus on Bone and Liver”. The article has undergone external peer review.
Reporting Checklist: The authors have completed the Narrative Review reporting checklist. Available at https://jlpm.amegroups.org/article/view/10.21037/jlpm-24-6/rc
Peer Review File: Available at https://jlpm.amegroups.org/article/view/10.21037/jlpm-24-6/prf
Conflicts of Interest: All authors have completed the ICMJE uniform disclosure form (available at https://jlpm.amegroups.org/article/view/10.21037/jlpm-24-6/coif). The series “Investigative Algorithms in Laboratory Medicine II: Focus on Bone and Liver” was commissioned by the editorial office without any funding or sponsorship. K.E.S. served as the unpaid Guest Editor of the series and serves as an unpaid editorial board member of the Journal of Laboratory and Precision Medicine from September 2022 to August 2024. N.J.L.G. declares consulting fees from Takeda Pharma, Ascendis Pharma and UCB Pharma, and serves as Chair for the Royal Osteoporosis Society and the Specialised Endocrinology Clinical Reference Group for National Health Service England Improvement, and as Global Co-Chair for the PARADIGHM patient registry for hypoparathyroidism (Takeda supported). The authors have no other conflicts of interest to declare.
Ethical Statement: The authors are accountable for all aspects of the work in ensuring that questions related to the accuracy or integrity of any part of the work are appropriately investigated and resolved.
Open Access Statement: This is an Open Access article distributed in accordance with the Creative Commons Attribution-NonCommercial-NoDerivs 4.0 International License (CC BY-NC-ND 4.0), which permits the non-commercial replication and distribution of the article with the strict proviso that no changes or edits are made and the original work is properly cited (including links to both the formal publication through the relevant DOI and the license). See: https://creativecommons.org/licenses/by-nc-nd/4.0/.
References
- Peacock M. Calcium metabolism in health and disease. Clin J Am Soc Nephrol 2010;5:S23-30. [Crossref] [PubMed]
- Portale AA, Perwad F. Calcium and Phosphorus. Pediatr Nephrol 2009;231-65.
- Veldurthy V, Wei R, Oz L, et al. Vitamin D, calcium homeostasis and aging. Bone Res 2016;4:16041. [Crossref] [PubMed]
- Jeon US. Kidney and calcium homeostasis. Electrolyte Blood Press 2008;6:68-76. [Crossref] [PubMed]
- Moor MB, Bonny O. Ways of calcium reabsorption in the kidney. Am J Physiol Renal Physiol 2016;310:F1337-50. [Crossref] [PubMed]
- Moe SM. Confusion on the complexity of calcium balance. Semin Dial 2010;23:492-7. [Crossref] [PubMed]
- Hill Gallant KM, Spiegel DM. Calcium Balance in Chronic Kidney Disease. Curr Osteoporos Rep 2017;15:214-21. [Crossref] [PubMed]
- Calcium [Internet]. The Association of UK Dieticians. Date accessed: 12/06/2023. Available online: https://www.bda.uk.com/resource/calcium.html
- McCabe LD, Martin BR, McCabe GP, et al. Dairy intakes affect bone density in the elderly. Am J Clin Nutr 2004;80:1066-74. [Crossref] [PubMed]
- Iuliano S, Poon S, Robbins J, et al. Effect of dietary sources of calcium and protein on hip fractures and falls in older adults in residential care: cluster randomised controlled trial. BMJ 2021;375: [Crossref] [PubMed]
- Al-Issa YAH. The Effect of Biological Treatment on Total and Ionized Calcium in Sera of Psoriasis Patients. Biochem Cell Arch 2020;20:1577-80.
- Gosling P. Analytical reviews in clinical biochemistry: calcium measurement. Ann Clin Biochem 1986;23:146-56. [Crossref] [PubMed]
- Robertson WG, Marshall RW. Calcium measurements in serum and plasma--total and ionized. CRC Crit Rev Clin Lab Sci 1979;11:271-304. [Crossref] [PubMed]
- Duvall LE, Shipman AR, Shipman KE. Investigative algorithms for disorders affecting plasma proteins with a focus on albumin and the calculated globulin fraction: a narrative review. J Lab Precis Med 2023;8:19. [Crossref]
- Wang S, McDonnell EH, Sedor FA, et al. pH effects on measurements of ionized calcium and ionized magnesium in blood. Arch Pathol Lab Med 2002;126:947-50. [Crossref] [PubMed]
- Bushinsky DA, Monk RD. Electrolyte quintet: Calcium. Lancet 1998;352:306-11. Erratum in: Lancet 2002;359:266. [Crossref] [PubMed]
- Hamroun A, Pekar JD, Lionet A, et al. Ionized calcium: analytical challenges and clinical relevance. J Lab Precis Med 2020;5:22. [Crossref]
- Pekar JD, Grzych G, Durand G, et al. Calcium state estimation by total calcium: the evidence to end the never-ending story. Clin Chem Lab Med 2020;58:222-31. [Crossref] [PubMed]
- Jassam N. Adjusted calcium equation: a step forward. J Lab Precis Med 2023;8:1. [Crossref]
- Jassam N, O’Kane M. Harmonisation of adjusted calcium equation, is it a realistic aim: a narrative review. J Lab Precis Med 2022;8:8. [Crossref]
- Roberts G, Thomas A. Standardisation of adjusted calcium equation: the UK approach—a narrative review. J Lab Precis Med 2022;8:4. [Crossref]
- Yap E, Goldwasser P. Can ionized calcium-estimating equations replace albumin-corrected calcium?—a narrative review. J Lab Precis Med 2022;7:13. [Crossref]
- Gernez E, Grzych G. Over what albumin concentration range are adjusted calcium equations valid? J Lab Precis Med 2022;7:28. [Crossref]
- Labriola L, Wallemacq P, Gulbis B, et al. The impact of the assay for measuring albumin on corrected (‘adjusted’) calcium concentrations. Nephrol Dial Transplant 2009;24:1834-8. [Crossref] [PubMed]
- Slomp J, van der Voort PH, Gerritsen RT, et al. Albumin-adjusted calcium is not suitable for diagnosis of hyper- and hypocalcemia in the critically ill. Crit Care Med 2003;31:1389-93. [Crossref] [PubMed]
- Glendenning P. It is time to start ordering ionized calcium more frequently: preanalytical factors can be controlled and postanalytical data justify measurement. Ann Clin Biochem 2013;50:191-3. [Crossref] [PubMed]
- Simons J. Correcting the Myth of Calcium Correction [Internet]. The University of British Columbia; 2019.
- Khundmiri SJ, Murray RD, Lederer E. PTH and Vitamin D. Compr Physiol 2016;6:561-601. [Crossref] [PubMed]
- Latic N, Erben RG. Interaction of Vitamin D with Peptide Hormones with Emphasis on Parathyroid Hormone, FGF23, and the Renin-Angiotensin-Aldosterone System. Nutrients 2022;14:5186. [Crossref] [PubMed]
- Tonon CR, Silva TAAL, Pereira FWL, et al. A Review of Current Clinical Concepts in the Pathophysiology, Etiology, Diagnosis, and Management of Hypercalcemia. Med Sci Monit 2022;28:e935821. [Crossref] [PubMed]
- Dent DM, Miller JL, Klaff L, et al. The incidence and causes of hypercalcaemia. Postgrad Med J 1987;63:745-50. [Crossref] [PubMed]
- Turner JJO. Hypercalcaemia - presentation and management. Clin Med (Lond) 2017;17:270-3. [Crossref] [PubMed]
- Walker MD, Shane E. Hypercalcemia: A Review. JAMA 2022;328:1624-36. [Crossref] [PubMed]
- Thongprayoon C, Cheungpasitporn W, Hansrivijit P, et al. Impact of Changes in Serum Calcium Levels on In-Hospital Mortality. Medicina (Kaunas) 2020;56:106. [Crossref] [PubMed]
- Minisola S, Pepe J, Piemonte S, et al. The diagnosis and management of hypercalcaemia. BMJ 2015;350:h2723. [Crossref] [PubMed]
- Lecoq AL, Livrozet M, Blanchard A, et al. Drug-Related Hypercalcemia. Endocrinol Metab Clin North Am 2021;50:743-52. [Crossref] [PubMed]
- Smit MA, van Kinschot CMJ, van der Linden J, et al. Clinical Guidelines and PTH Measurement: Does Assay Generation Matter? Endocr Rev 2019;40:1468-80. [Crossref] [PubMed]
- Christensen SE, Nissen PH, Vestergaard P, et al. Familial hypocalciuric hypercalcaemia: a review. Curr Opin Endocrinol Diabetes Obes 2011;18:359-70. [Crossref] [PubMed]
- Bollerslev J, Rejnmark L, Zahn A, et al. European Expert Consensus on Practical Management of Specific Aspects of Parathyroid Disorders in Adults and in Pregnancy: Recommendations of the ESE Educational Program of Parathyroid Disorders. Eur J Endocrinol 2022;186:R33-63. [Crossref] [PubMed]
- Lewis R. Mineral and bone disorders in chronic kidney disease: new insights into mechanism and management. Ann Clin Biochem 2012;49:432-40. [Crossref] [PubMed]
- Zhang LX, Zhang B, Liu XY, et al. Advances in the treatment of secondary and tertiary hyperparathyroidism. Front Endocrinol (Lausanne) 2022;13:1059828. [Crossref] [PubMed]
- Mirrakhimov AE. Hypercalcemia of Malignancy: An Update on Pathogenesis and Management. N Am J Med Sci 2015;7:483-93. [Crossref] [PubMed]
- Horwitz MJ, Tedesco MB, Sereika SM, et al. Continuous PTH and PTHrP infusion causes suppression of bone formation and discordant effects on 1,25(OH)2 vitamin D. J Bone Miner Res 2005;20:1792-803. [Crossref] [PubMed]
- Rajkumar SV, Dimopoulos MA, Palumbo A, et al. International Myeloma Working Group updated criteria for the diagnosis of multiple myeloma. Lancet Oncol 2014;15:e538-48. [Crossref] [PubMed]
- Kazandjian D. Multiple myeloma epidemiology and survival: A unique malignancy. Semin Oncol 2016;43:676-81. [Crossref] [PubMed]
- Zagzag J, Hu MI, Fisher SB, et al. Hypercalcemia and cancer: Differential diagnosis and treatment. CA Cancer J Clin 2018;68:377-86. [Crossref] [PubMed]
- Koulouri O, Gurnell M. How to interpret thyroid function tests. Clin Med (Lond) 2013;13:282-6. [Crossref] [PubMed]
- Michels A, Michels N. Addison disease: early detection and treatment principles. Am Fam Physician 2014;89:563-8. [PubMed]
- Flowers KC, Allen GT, Darragh-Hickey C, et al. Investigative algorithms for disorders affecting plasma potassium: a narrative review. J Lab Precis Med 2022;7:25. [Crossref]
- Marcinowska-Suchowierska E, Kupisz-Urbańska M, Łukaszkiewicz J, et al. Vitamin D Toxicity-A Clinical Perspective. Front Endocrinol (Lausanne) 2018;9:550. [Crossref] [PubMed]
- Lee JP, Tansey M, Jetton JG, et al. Vitamin D Toxicity: A 16-Year Retrospective Study at an Academic Medical Center. Lab Med 2018;49:123-9. [Crossref] [PubMed]
- Sharma OP. Hypercalcemia in granulomatous disorders: a clinical review. Curr Opin Pulm Med 2000;6:442-7. [Crossref] [PubMed]
- Ungprasert P, Ryu JH, Matteson EL. Clinical Manifestations, Diagnosis, and Treatment of Sarcoidosis. Mayo Clin Proc Innov Qual Outcomes 2019;3:358-75. [Crossref] [PubMed]
- Eurelings LEM, Miedema JR, Dalm VASH, et al. Sensitivity and specificity of serum soluble interleukin-2 receptor for diagnosing sarcoidosis in a population of patients suspected of sarcoidosis. PLoS One 2019;14:e0223897. [Crossref] [PubMed]
- Grosso S, Margollicci MA, Bargagli E, et al. Serum levels of chitotriosidase as a marker of disease activity and clinical stage in sarcoidosis. Scand J Clin Lab Invest 2004;64:57-62. [Crossref] [PubMed]
- Narasimhan P, Wood J, Macintyre CR, et al. Risk factors for tuberculosis. Pulm Med 2013;2013:828939. [Crossref] [PubMed]
- Coker R, McKee M, Atun R, et al. Risk factors for pulmonary tuberculosis in Russia: case-control study. BMJ 2006;332:85-7. [Crossref] [PubMed]
- Abdulgader SM, Okunola AO, Ndlangalavu G, et al. Diagnosing Tuberculosis: What Do New Technologies Allow Us to (Not) Do? Respiration 2022;101:797-813. [Crossref] [PubMed]
- Zayed RF, Millhouse PW, Kamyab F, et al. Calcium-Alkali Syndrome: Historical Review, Pathophysiology and Post-Modern Update. Cureus 2021;13:e13291. [Crossref] [PubMed]
- Cerqueira A, Seco T, Costa A, et al. Pheochromocytoma and Paraganglioma: A Review of Diagnosis, Management and Treatment of Rare Causes of Hypertension. Cureus 2020;12:e7969. [Crossref] [PubMed]
- Katreddy VM, Ashawesh K, Saraf S, et al. Unusual cause of hyercalcemia in pheochromocytoma. Endocr Abstr 2012;22:450.
- Danese E, Montagnana M, Brentegani C, et al. Short-term stability of free metanephrines in plasma and whole blood. Clin Chem Lab Med 2020;58:753-7. [Crossref] [PubMed]
- Patel C, Anbari RA, Lomonaco MR, Hypervitaminosis A. A Rare Cause of Hypercalcemia. J Endocr Soc 2021;5:A199-200. [Crossref]
- Farrington K, Miller P, Varghese Z, et al. Vitamin A toxicity and hypercalcaemia in chronic renal failure. Br Med J (Clin Res Ed) 1981;282:1999-2002. [Crossref] [PubMed]
- Karakosta T, Wan Y, Truong D. Establishing preanalytical stability of vitamin A and vitamin E. Clin Biochem 2023;115:144-8. [Crossref] [PubMed]
- Tettero JM, van Eeghen E, Kooter AJ. Extreme hypercalcaemia caused by immobilisation due to acute spinal cord injury. BMJ Case Rep 2021;14:e241386. [Crossref] [PubMed]
- Cooper MS, Gittoes NJ. Diagnosis and management of hypocalcaemia. BMJ 2008;336:1298-302. [Crossref] [PubMed]
- Hannan FM, Thakker RV. Investigating hypocalcaemia. BMJ 2013;346:f2213. [Crossref] [PubMed]
- Davidson DF. Effects of contamination of blood specimens with liquid potassium-EDTA anticoagulant. Ann Clin Biochem 2002;39:273-80. [Crossref] [PubMed]
- Sharratt CL, Gilbert CJ, Cornes MC, et al. EDTA sample contamination is common and often undetected, putting patients at unnecessary risk of harm. Int J Clin Pract 2009;63:1259-62. [Crossref] [PubMed]
- Liamis G, Milionis HJ, Elisaf M. A review of drug-induced hypocalcemia. J Bone Miner Metab 2009;27:635-42. [Crossref] [PubMed]
- Hassan-Smith Z, Gittoes N. Hypocalcaemia. Med (United Kingdom) 2017;45:555-9.
- Bilezikian JP, Khan A, Potts JT Jr, et al. Hypoparathyroidism in the adult: epidemiology, diagnosis, pathophysiology, target-organ involvement, treatment, and challenges for future research. J Bone Miner Res 2011;26:2317-37. [Crossref] [PubMed]
- Vetter T, Lohse MJ. Magnesium and the parathyroid. Curr Opin Nephrol Hypertens 2002;11:403-10. [Crossref] [PubMed]
- Darragh-Hickey C, Kaur S, Flowers KC, et al. Investigative algorithms for disorders affecting plasma magnesium: a narrative review. J Lab Precis Med 2022;7:21. [Crossref]
- Linglart A, Levine MA, Jüppner H. Pseudohypoparathyroidism. Endocrinol Metab Clin North Am 2018;47:865-88. [Crossref] [PubMed]
- Sury K. Update on the prevention and treatment of tumor lysis syndrome. J Onco-Nephrology 2019;3:19-30. [Crossref]
- Waldron JL, Ashby HL, Cornes MP, et al. Vitamin D: a negative acute phase reactant. J Clin Pathol 2013;66:620-2. [Crossref] [PubMed]
- Sanders KM, Stuart AL, Williamson EJ, et al. Annual high-dose oral vitamin D and falls and fractures in older women: a randomized controlled trial. JAMA 2010;303:1815-22. [Crossref] [PubMed]
- Woodford HJ, Barrett S, Pattman S. Vitamin D: too much testing and treating? Clin Med (Lond) 2018;18:196-200. [Crossref] [PubMed]
- Lee JH, Choi JH, Kweon OJ, et al. Discrepancy between Vitamin D Total Immunoassays due to Various Cross-reactivities. J Bone Metab 2015;22:107-12. [Crossref] [PubMed]
- Goff JP, Reinhardt TA, Engstrom GW, et al. Effect of dietary calcium or phosphorus restriction and 1,25-dihydroxyvitamin D administration on rat intestinal 24-hydroxylase. Endocrinology 1992;131:101-4. [Crossref] [PubMed]
- Ahmed A, Azim A, Gurjar M, et al. Hypocalcemia in acute pancreatitis revisited. Indian J Crit Care Med 2016;20:173-7. [Crossref] [PubMed]
- Ismail OZ, Bhayana V. Lipase or amylase for the diagnosis of acute pancreatitis? Clin Biochem 2017;50:1275-80. [Crossref] [PubMed]
- Byerly S, Inaba K, Biswas S, et al. Transfusion-Related Hypocalcemia After Trauma. World J Surg 2020;44:3743-50. [Crossref] [PubMed]
- Kumar A, Kaur S. Calcium: A Nutrient in Pregnancy. J Obstet Gynaecol India 2017;67:313-8. [Crossref] [PubMed]
- Kovacs CS. Fuleihan Gel-H. Calcium and bone disorders during pregnancy and lactation. Endocrinol Metab Clin North Am 2006;35:21-51. v. [Crossref] [PubMed]
- Hofmeyr GJ, Lawrie TA, Atallah ÁN, et al. Calcium supplementation during pregnancy for preventing hypertensive disorders and related problems. Cochrane Database Syst Rev 2018;10:CD001059. [Crossref] [PubMed]
- Rodd C, Goodyer P. Hypercalcemia of the newborn: etiology, evaluation, and management. Pediatr Nephrol 1999;13:542-7. [Crossref] [PubMed]
- Improda N, Mazzeo F, Rossi A, et al. Severe hypercalcemia associated with hypophosphatemia in very premature infants: a case report. Ital J Pediatr 2021;47:155. [Crossref] [PubMed]
- Samedi VM, Yusuf K, Yee W, et al. Neonatal hypercalcemia secondary to subcutaneous fat necrosis successfully treated with pamidronate: a case series and literature review. AJP Rep 2014;4:e93-6. [Crossref] [PubMed]
Cite this article as: Flowers KC, Shipman KE, Shipman AR, Gittoes NJL. Investigative algorithms for disorders causing hypercalcaemia and hypocalcaemia: a narrative review. J Lab Precis Med 2024;9:27.