Investigative algorithms for disorders causing hypophosphataemia and hyperphosphataemia: a narrative review
IntroductionOther Section
- Introduction
- Methods
- Background
- Hypophosphataemia
- Hyperphosphataemia
- Special states
- Conclusions
- Acknowledgments
- Footnote
- References
Phosphate is the most abundant anion in the human body (1) and makes up approximately 1% of total body weight in adults (2). Phosphate has essential roles in energy metabolism and ribonucleic acid structure, and it is one of the most important contributors to overall bone health (3). Although phosphate is 100× more concentrated in the intracellular compartment compared to the extracellular compartment (4), phosphate is commonly measured in plasma or serum as part of a ‘bone profile’. Phosphate homeostasis in humans is complex (5), but it is important to understand the basics to be able to elucidate the principal causes of abnormal phosphate concentrations in the blood.
The following article presents algorithms designed to provide a systematic approach to the diagnostic work up of a patient with hypo- or hyperphosphataemia. These algorithms will not address treatments and are not designed to replace local guidelines or specialist endocrinological knowledge/advice. The reader is still encouraged to refer more complex and/or rare phosphate abnormalities to specialist departments for appropriate investigation and treatment, particularly if a genetic condition in a child is suspected. Instead, these algorithms are most useful as entry level general diagnostic aids to identify common causes of phosphate derangements. Important laboratory and analytical considerations, which are frequently encountered in routine practice but are often not mentioned in guidelines and other reviews, will also be discussed. For conditions where the primary clinical abnormality usually causes a concurrent calcium disturbance—e.g., parathyroid disease—the reader is directed to the companion article in this series on calcium (6), and to other comprehensive open-access clinical guidelines (7). We present this article in accordance with the Narrative Review reporting checklist (available at https://jlpm.amegroups.org/article/view/10.21037/jlpm-24-35/rc).
MethodsOther Section
- Introduction
- Methods
- Background
- Hypophosphataemia
- Hyperphosphataemia
- Special states
- Conclusions
- Acknowledgments
- Footnote
- References
The literature review was created by searching PubMed, Medline, OMIM and Google Scholar. The diagnostic algorithms were then created using the information gathered from the literature review as well as the expert opinion of the authors. Literature was searched over the period November 2023 to March 2024 and language was restricted to English (see Table 1 for further information).
Table 1
Items | Specification |
---|---|
Date of search | November 2023 to March 2024 |
Databases and other sources searched | PubMed, Medline, Google Scholar, OMIM |
Search terms used | Phosphate, hypophosphataemia, hyperphosphataemia, drugs, investigations, algorithms, guidelines, diagnosis, causes, aetiology, human |
Timeframe | Database inception to March 2024 |
Inclusion criteria | All papers and reviews were included, but language was restricted to English |
Selection process | A.R.S. and K.E.S. conducted initial search, with most of the refinement and technical additions done by K.C.F. and N.J.L.G. All authors obtained consensus and agreement |
Any additional considerations, if applicable | Seminal texts were also searched and the references of important articles and texts were obtained and checked for relevance |
BackgroundOther Section
- Introduction
- Methods
- Background
- Hypophosphataemia
- Hyperphosphataemia
- Special states
- Conclusions
- Acknowledgments
- Footnote
- References
Phosphorus and phosphate terminology and types
The terms “phosphorus” and “phosphate” are frequently used interchangeably throughout the medical literature. Technically however, they are different because phosphorus refers to the phosphorus atom (P) only, whereas phosphate is a compound comprising of one phosphorus atom and four oxygen atoms (PO43−) (4). This technicality is not clinically important when the international system of units (SI) (common in Europe) are used—which express the phosphorus concentration as the number of particles per unit of volume—because one mole of phosphate contains one mole of phosphorus (4,8). Importantly however, this difference is certainly relevant if conventional mass units (common in the United States) are used—which express phosphorus concentration as the mass per unit of volume—where the extra weight of the additional oxygen atoms in phosphate has a significant effect (9,10). For example, a phosphorus (P) concentration of 4 mmol/L represents a phosphate (PO43−) concentration of 4 mmol/L. Conversely, a phosphorus (P) concentration of 4 mg/dL represents a phosphate (PO43−) concentration of 12.3 mg/dL as the molar mass of phosphate (94.97 g/mol) is 3.07 times the molar mass of phosphorus (30.97 g/mol) (4). It is, therefore, important that the correct “phosphate” or “phosphorus” term is used if conventional mass units are utilised, especially when converting from SI units where either term is accurate. See Figure 1 for a summary of the difference between phosphorus and phosphate as well as approximate conversion factors. For consistency and clarity, the term “phosphate” expressed in mmol/L will be used throughout this review, and conversions to mg/dL units will be to “phosphorus”.
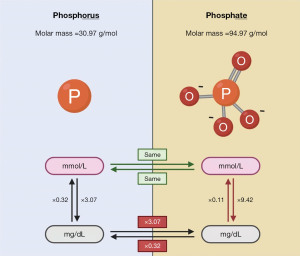
Elemental phosphorus is very reactive and does not exist by itself in nature (4). Therefore, in the human body, phosphorus exists as part of two forms of phosphate: organic and inorganic. Organic phosphate is mostly bound to proteins and carbon containing molecules, and high amounts are found naturally in protein rich food (9). Approximately 30% to 60% of dietary organic phosphorus is hydrolysed and absorbed into the circulation as inorganic phosphate (Pi) (9). Direct dietary sources of Pi include preservatives and added salts in ultra-processed foods (9). Dietary Pi can significantly increase phosphate intake as conversely to organic phosphate, Pi are easily dissociable salts, meaning that more than 90% is absorbed in the gastrointestinal tract (9).
Pi in blood is 85% free (PO43−), 10% bound to plasma proteins and 5% complexed with calcium, magnesium and sodium (4). The free Pi form is the form measured by laboratory methods and is the biologically active form that is the driver of homeostatic mechanisms (8).
Phosphate functions and balance
Important functions of phosphate include: part of adenosine di- and tri-phosphate (ADP and ATP) that are the main energy molecules in humans; part of nicotine adenine diphosphate (NADP) that is a buffer and enzyme activator; part of cell signalling molecules such as cyclic adenosine or guanosine monophosphate (cAMP or cGMP); and an oxygen transporter (5). Phosphate is also important in bone and teeth maintenance (and structure) as well as muscle function (11). The normal reference interval for phosphate in blood for adults is approximately 0.80 to 1.45 mmol/L (2.48 to 4.50 mg/dL) (8). Most (85%) of the total body phosphate is stored in bones and teeth (11), therefore plasma phosphate concentrations may not reflect the true body store of phosphate.
Figure 2 shows the phosphate balance in adults; values are approximated for a 75 kg person (3,5). Children have a positive balance of 2–3 mmol/day (6.2–9.3 mg/day) of phosphate as they increase the phosphate stored in their bones and teeth (5). There is negative balance later in life due to age related bone loss (5) and a higher risk of dietary deficiency and/or decreased absorption, which may lead to osteomalacia (11).
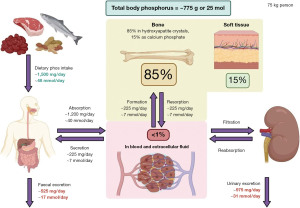
Phosphate homeostasis
The homeostatic regulation of phosphate is complex due to the number of different hormones and transporters that are active at the same time. A full review of the most up to date insights into phosphate homeostasis is beyond the scope of this article; the reader is directed to other comprehensive reviews if more information is required (5,11), particularly the recent review by Levi et al. (12) and the seminal review of renal regulation by Farrow and White (2). However, a simplified overview will be provided here to provide context for interpreting phosphate results in clinical practice.
The major hormones involved in phosphate metabolism are parathyroid hormone (PTH), 1,25-dihydroxyvitamin D, and fibroblast growth factor 23 (FGF-23) (11). Other important hormones include insulin, ectonucleotide pyrophosphatase/phosphodiesterase-1 (ENPP1) (13), growth hormone, adrenaline (epinephrine), thyroid hormones and steroids (12).
PTH
The primary function of PTH is to regulate blood ionised (free) calcium concentration, where hypocalcaemia stimulates PTH release from the parathyroid glands (14). The phosphate relevant renal effects of increased PTH action is the endocytosis and destruction of sodium-phosphate co-transporters type 2a (NPT2a) in the proximal tubules, resulting in reduced phosphate reabsorption from the renal filtrate and hence increased urinary excretion (2). The importance of the effect of PTH on phosphate homeostasis is evident in patients with primary hyperparathyroidism (high PTH) where there is renal phosphate wasting and possible hypophosphataemia. In hypoparathyroidism (low PTH), there is increased renal phosphate reabsorption and possible hyperphosphataemia (2).
PTH also upregulates the renal enzyme 1α-hydroxylase, which in turn converts inactive 25-hydroxyvitamin D to active 1,25-dihydroxyvitamin D (14). 1,25-dihydroxyvitamin D increases phosphate and calcium absorption from the gut to allow an increase in blood calcium whilst keeping blood phosphate stable (2,14). Therefore, a high 1,25-dihydroxyvitamin D in hypophosphataemia is expected in health in an attempt to increase gut absorption.
It should be noted that the routine immunoassay tests used to measure PTH can cross react with biologically inactive fragments and overestimate the concentration, particularly in patients with chronic kidney disease (CKD). Consequently, clinicians should ensure that the test used in their local laboratory is at least a second (preferably third) generation immunoassay, especially if the PTH result is causing diagnostic confusion [see Smit et al. (15), or the calcium article within this series (6), for more information on PTH testing].
FGF-23
FGF-23 is primarily released from osteocytes and osteoblasts in bone tissue in response to increased phosphate and 1,25-dihydroxyvitamin D in the blood (16). Similarly to PTH, FGF-23 down regulates NPT2a and NPT2c in the kidney to increase phosphate excretion in the urine (16). FGF-23 exerts these effects in the kidney via FGF receptors and its co-factor α-Klotho, and phosphate homeostasis disorders can manifest if these do not function correctly (16,17).
FGF-23 may also be useful as a marker of bone disease and a very early marker of CKD (18). There are strong associations between high levels of circulating FGF-23 and multiple cardiovascular (CV) abnormalities (16). However, a very recent large study found no association between increased CV abnormalities and genetically predicted FGF-23 concentration, suggesting that there is indeed no important causal link that would make targeting FGF-23 an effective CV disease treatment (19).
FGF-23 and PTH are both key to phosphate metabolism, and excess or deficiency of either result in hypophosphataemia and hyperphosphataemia respectively (20). However, PTH increases the production of active 1,25-dihydroxyvitamin D whilst FGF-23 decreases the production (21). See Figure 3 for a simplified overview of the main effects of PTH, FGF-23 and vitamin D on phosphate regulation in the kidneys and the gut (2,5,22).
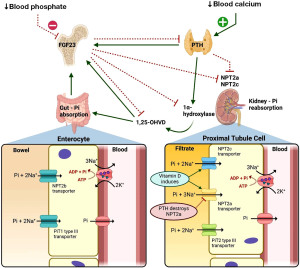
Diurnal variation
An important but often forgotten property of blood phosphate concentration is its diurnal variation. Blood phosphate is known to be at its lowest point—approximately 1.07 to 1.16 mmol/L (3.32 to 3.60 mg/dL)—between 08:00 and 11:00, and at its highest point—≥1.49 mmol/L (≥4.62 mg/dL)—between 02:00 and 04:00 (23). This circadian variation is also present to a similar degree in patients with end stage renal disease (24), but fasting for 96 hours blunts this circadian variation (25). It may, therefore, be appropriate to take a blood sample for phosphate measurement at a different time of day than a sample that showed a borderline abnormal phosphate concentration, or after a period of fasting (if safe to do so), to confirm a genuine phosphate imbalance.
Fasting state
Compared to baseline concentrations, plasma phosphate concentration decreases by approximately 4% (26) to 8% (27) one hour after a meal, and then increases by approximately 5% (27) to 12% (26) four hours after a meal. Consequently, patients who have a borderline low or high phosphate concentration in the blood should have a fasting phosphate measured to confirm the abnormal phosphate status at baseline. However, significant phosphate abnormalities, especially in conjunction with other clinically relevant factors, are unlikely going to be explained by non-clinically significant post-prandial variation. The proposed investigative algorithms below will assume that abnormal phosphate concentrations are present in samples taken during fasting,
HypophosphataemiaOther Section
- Introduction
- Methods
- Background
- Hypophosphataemia
- Hyperphosphataemia
- Special states
- Conclusions
- Acknowledgments
- Footnote
- References
Hypophosphataemia can be defined as a plasma phosphate concentration <0.80 mmol/L (<2.48 mg/dL) (5), although this may vary slightly depending on the local population and the laboratory method utilised. Biochemically moderate hypophosphataemia is generally considered to be between 0.31 and 0.50 mmol/L (0.96 and 1.55 mg/dL) (28), and biochemically severe hypophosphataemia is ≤0.30 mmol/L (≤0.93 mg/dL) (5). Importantly however, these crude biochemical categorisations may not reflect the clinical significance of hypophosphataemia, therefore clinicians must consider other factors—including the speed of decrease and other relevant pathologies—when determining the clinical urgency.
Biochemically low phosphate may be present in approximately 11% of all patients who have blood phosphate analysis requested (28). Almost all (99%) samples from patients with moderate to severe hypophosphataemia (≤0.5 mmol/L) come from hospitalised patients, and patients in intensive care units make up 45% of this hospitalised patient group (28). There is a higher incidence of hypophosphataemia in those who are critically ill (29), and it is associated with poorer outcomes in hospitalised patients with coronavirus disease 2019 (COVID-19) (30). The commonest causes and clinical presentations associated with hypophosphataemia in hospitalised patients are (28,31-34):
- Unwell enough to be in intensive care (and more frequent in the sickest of intensive care unit patients);
- Neoplasms;
- Refeeding syndrome (RFS);
- Sepsis;
- Gastrointestinal loss;
- Intravenous fluid replacement (non-phosphate containing);
- Diabetic ketoacidosis (DKA);
- Excessive alcohol intake;
- Mechanical ventilation.
Symptoms of hypophosphataemia can be non-specific but can be life-threatening, and include muscle weakness, impaired myocardial contractility and ventricular arrhythmias, respiratory failure, rhabdomyolysis, ileus, immune dysfunction and encephalopathy (35). A proposed algorithm for the laboratory investigation of hyperphosphataemia is shown in Figure 4.
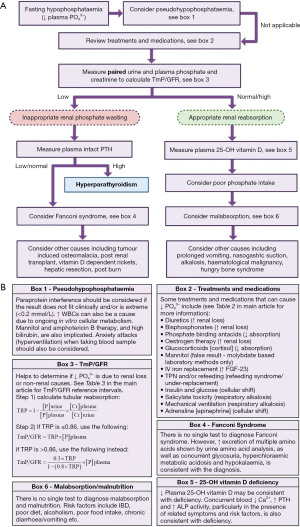
Pseudohypophosphataemia
The possibility of a falsely low plasma phosphate result should always be considered in order to prevent unnecessary investigation and treatment (36), especially if the result is unexpected due to an absence of symptoms or risk factors, and/or the results do not respond to treatment.
Although more commonly associated with pseudohyperphosphataemia, the presence of a paraprotein in lymphoproliferative disorders—including multiple myeloma, Waldenstrom macroglobulinaemia and lymphoma—can cause falsely low phosphate results due to interference with the laboratory method (37-39). If suspected, serum protein electrophoresis, and urine protein electrophoresis or serum free light chains, should be requested to identify potential interfering paraproteins (40). If present, paraprotein interference can be confirmed by analysis using a different laboratory method, where a significantly different result is suspicious. If this is not possible, precipitation of plasma/serum using polyethylene glycol (PEG) can remove the interfering paraprotein and provide a more reliable result, a method which has been successfully used to confirm paraprotein interference in other analytes (41).
Falsely low phosphate (and potassium) results have also been reported in samples from patients with extreme leucocytosis, which can be confirmed by a subsequent normal phosphate after rapid analysis on a fresh sample transported to the laboratory on ice (42). The exact mechanism is uncertain, but it is possible that the continued metabolism of leucocytes in vitro expend extracellular phosphate in the sample before separation of serum/plasma from the cells occurs in the laboratory (42).
High bilirubin concentrations can cause falsely low phosphate results in biochromatic phosphomolybdate methods, but the use of a reducing agent can negate this effect (43). Mannitol treatment can cause pseudohypophosphataemia by binding to molybdate in the laboratory method (44-46), causing a decreased rate of colour development and a lower endpoint measurement. However, mannitol therapy can also contribute to genuine hypophosphataemia as it has an osmotic effect and mildly increases phosphate excretion in the urine (32,47). Finally, falsely low phosphate results has also been demonstrated in patients receiving high dose antifungal liposomal amphotericin B therapy (48).
Hyperventilation due to anxiety and/or needle phobic panic attacks can cause a respiratory alkalosis, which in turn can cause hypophosphataemia. Although this is technically a true hypophosphataemia, this is usually mild and not clinically significant, and usually does not require further investigation if no other relevant clinical factors are present (49).
Treatments and medications
Once pseudohypophosphataemia has been excluded, known treatments and medications should be reviewed to determine if these may be causing/contributing to the genuine hypophosphataemia [see Table 2, and Figure 4B box 2, for a non-exhaustive list of medications and treatments that are associated with hypophosphataemia (47,50-52)]. Many cancer therapies have been implicated in drug induced hypophosphataemia; see the comprehensive review by Adhikari et al. (32) for more information. Once identified, it may be appropriate to stop the relevant medication(s), review the dose or just monitor depending on the severity of hypophosphataemia, requirement of the medication, and availability of alternatives.
Table 2
Mechanism of action | Examples of drugs/therapies |
---|---|
Pseudohypophosphataemia | Mannitol, liposomal amphotericin B |
Proximal tubule injury (drug induced Fanconi syndrome) | Chemotherapy, e.g., cisplatin, ifosfamide, alkylating agents. New classes including nivolumab, ipilimumab, imatinib, vemurafenib |
Hypomagnesaemia and hypocalcaemia leading to vitamin D resistance and PTH secretion | Antimetabolite agents, azacitidine, antacids |
Inhibition of PDGF receptor expressed in bone and proximal tubular cells | Imatinib or sunitinib |
Vitamin D deficiency/resistance | Sorafenib, phenytoin, phenobarbital |
Inhibition of IGF-1 receptor in proximal tubule | Ceritinib |
Downregulation of type 2a Na+–phosphate cotransporter in proximal tubule | Oestrogen |
Reduction of intestinal absorption | Antacids, glucocorticoids, elemental feeds, PPIs, phosphate binders (e.g., aluminium hydroxide), laxatives, calcium supplements |
Increased concentration of FGF-23 | Iron infusions (particularly FCM) |
Shift of phosphate into cells | Salicylic acid poisoning (leading to respiratory acidosis), glucose, dextrose, fructose, insulin, parenteral nutrition, catecholamines, β-adrenergic drugs (e.g., salbutamol or theophylline), EPO and GM-CSF (causing cell proliferation). Likely cause in neuroleptic malignant syndrome (rare complication of typical and atypical antipsychotic drugs) |
Increased urinary phosphate excretion | Carbonic anhydrase inhibitors, diuretics (hydochlorthiazide, furosemide), theophylline, bronchodilators, corticosteroids, bisphosphonates, aciclovir |
Drug induced metabolic acidosis | Alcohol and toluene |
Unknown | Paracetamol (acetaminophen) |
PTH, parathyroid hormone; PDGF, platelet derived growth factor; IGF-1, insulin-like growth factor 1; PPIs, proton pump inhibitors; FGF-23, fibroblast growth factor 23; FCM, ferric carboxymaltose; EPO, erythropoietin; GM-CSF, granulocyte-macrophage colony-stimulating factor.
If no known hypophosphataemia causing medications are being taken, the degree of hypophosphataemia is not consistent with the particular medication, or hypophosphataemia persists when the drug is discontinued or the dose is reduced, then further investigation is likely appropriate.
Iron infusion (intravenous)
A particularly topical and relatively recently appreciated cause of hypophosphataemia is iron infusion (intravenous). The main mechanism is due to increased urinary phosphate excretion as a consequence of increased concentrations of full length biologically active FGF-23 (53), which increases three to six fold within the first day of infusion (54). The increased FGF-23 activity additionally decreases 1,25-dihydroxyvitmain D (53), resulting in the compounding effect of reducing phosphate and calcium absorption from the diet and secondary hyperparathyroidism. Iron infusion-induced hypophosphataemia can be severe enough to cause fragility fractures and symptomatic osteomalacia (54-57).
The particular formulation of iron infusion significantly affects the risk of iron induced hypophosphataemia. The highest risk is associated with ferric carboxymaltose (FCM) (Ferinject®, Injectafer®)—which is associated with an incidence of hypophosphataemia of 47% to 75%—compared to an incidence of <10% with ferric derisomaltose (FDI) (formerly known as iron isomaltoside 1000), ferumoxytol (FMX) (Feraheme®) and low molecular weight iron dextran (LMWID) (INFeD®, Cosmofer®) (53). Blood phosphate concentrations are usually lowest two weeks after FCM administration, and hypophosphataemia can be difficult to correct and can persist for weeks to several months (54). Risk factors for severe iron infusion-induced hypophosphataemia include lower baseline serum phosphate, normal kidney function (poor kidney function reduces renal loss), severe iron deficiency, lower body weight and repeated doses of iron infusion (53). See Martens and Wolf (53) for more information.
Urine phosphate measurement—tubular maximum reabsorption [tubular maximum reabsorption rate of phosphate to glomerular filtration rate (TmP/GFR)]
The concentration of phosphate in the urine depends on the plasma concentration and the glomerular filtration rate (GFR), assuming the renal tubules are working maximally. A crude cut-off of <1 mmol/L of phosphate in urine (from a 24-hour collection) during hypophosphataemia can be used to suggest that renal loss is not the cause of hypophosphataemia (58). However, during hypophosphataemia, it is generally more reliable to calculate the TmP/GFR to estimate if the urinary phosphate indicates inappropriate renal loss (phosphate wasting from renal causes) or appropriate renal reabsorption (non-renal causes) (59).
To calculate the TmP/GFR, a second void early morning random/spot urine sample, and a plasma sample taken at a similar time, should both be analysed for phosphate and creatinine. There are two steps to the calculation. Firstly, calculate the tubular reabsorption of phosphate (TRP) using the equation in Figure 4B box 3. If the TRP is ≤0.86, the renal reabsorption of phosphate is maximal and there is a linear relationship between plasma phosphate concentration and urinary excretion, and the TmP/GFR is simply calculated by multiplying the TRP by the plasma phosphate concentration. However, if the TRP is >0.86, the renal reabsorption of phosphate is not maximal and there is a curvilinear relationship between plasma phosphate concentration and urinary excretion. A different equation needs to be used to calculate TmP/GFR in these situations (see Figure 4B box 3) (59). If phosphate is measured in mmol/L, the TmP/GFR is expressed as mmol/L of glomerular filtrate.
Table 3 displays age-related reference intervals for TmP/GFR (59). If the TmP/GFR is less than the age related reference interval, inappropriate renal phosphate wasting is suggested, and renal causes of hypophosphataemia should be explored (59). Normal/high TmP/GFR suggest appropriate renal reabsorption of phosphate, and non-renal causes are more likely.
Table 3
Age | Range |
---|---|
0 to <3 months | 1.43–3.43 |
3 to <6 months | 1.48–3.30 |
6 months to <2 years | 1.15–2.60 |
2 to 15 years | 1.15–2.44 |
25 to 35 years | |
Male | 1.00–1.35 |
Female | 0.96–1.44 |
45 to 55 years | |
Male | 0.90–1.35 |
Female | 0.88–1.42 |
65 to75 years | |
Male | 0.8–1.35 |
Female | 0.80–1.35 |
From Payne (59). TmP/GFR, tubular maximum reabsorption rate of phosphate to glomerular filtration rate.
High urinary phosphate excretion (low TmP/GFR)
High urinary phosphate excretion (low TmP/GFR) in the presence of hypophosphataemia (indicating renal phosphate wasting) demonstrates that physiologically inappropriate renal handling of phosphate is the likely cause. DKA should always be considered and ruled out due to the clinical urgency. DKA causes hypophosphataemia due to osmotic diuresis from renal glucose excretion, leading to increased urinary phosphate excretion. Treatment with insulin can exacerbate the low phosphate due to the shift from the extracellular to the intracellular compartment. DKA has not been included in the algorithm in Figure 4 as the clinical presentation is unlikely to cause diagnostic confusion (60).
PTH analysis is a useful next step to determine if the inappropriate renal phosphate wasting is caused by hyperparathyroidism or something else. Hyperparathyroidism (primary and tertiary) is an important and common cause of renal phosphate wasting, detected by inappropriately normal or high PTH concentrations in the presence of hypercalcaemia. Hyperparathyroidism has been covered elsewhere; the reader is recommended to review the companion article in this series on calcium (6) and other sources for a full discussion (7). Secondary hyperparathyroidism is notable as this diagnosis of exclusion is defined as a persistently elevated PTH concentration in the presence of normocalcaemia (7). Hyperphosphataemia (61) and FGF-23 dependent (62) hypophosphataemia stimulate PTH secretion, therefore phosphate metabolism issues may be the cause of secondary hyperparathyroidism. If the primary cause of secondary hyperparathyroidism is not hyperphosphataemia (i.e., renal failure causes less excretion of phosphate and PTH secretion is increased to compensate), hypophosphataemia may be a consequence of the secondary hyperparathyroidism from other causes instead (e.g., due to calcium/vitamin D deficiency).
Fanconi syndrome
Fanconi syndrome is a general dysfunction of renal proximal tubules, leading to excessive excretion (wasting) of amino acids, phosphate, glucose, bicarbonate, uric acid and other solutes (63). Fanconi syndrome in children can lead to excess phosphate loss and cause rickets. See Table 4 for a non-exhaustive summary of inherited causes (which often present in young patients) and acquired causes of Fanconi syndrome (63). Due to its syndromic nature, there is no single test to diagnose Fanconi syndrome, which is often diagnosed when one of the conditions in Table 4 are diagnosed or met. Urine amino acid analysis can be helpful to demonstrate excess urinary excretion of multiple amino acids, which may be consistent with Fanconi syndrome. Other findings which may suggest Fanconi syndrome in the appropriate clinical context include hypokalaemia, glycosuria and hyperchloraemic metabolic acidosis (63).
Table 4
Inherited | Acquired |
---|---|
Wilson disease (↓ plasma copper, ↓ plasma caeruloplasmin, ↑ urinary copper excretion) (63) | Excessive immunoglobin light chain production in multiple myeloma, amyloidosis etc. (SPE, and UPE or SFLCs) (64) |
Cystinosis (↑ intracellular cysteine in WBCs) (65) | Renal transplant |
Hereditary fructose intolerance (ALDOB genomic analysis, aldolase B activity on liver biopsy) (66) | Nephrotic syndrome (↓ plasma albumin, ↑ plasma lipids, ↑ urinary protein:creatinine ratio) |
Galactosaemia (↓ Gal-1-PUT in RBCs, GALT/GALE/GALK genomic analysis) (67) | Drugs: cisplatin, gentamicin, azathioprine, valproate, etc. (62) |
Tyrosinaemia (↑ blood succinylacetone) (68,69) | Toluene exposure [glue sniffing] (70) |
Glycogen storage disease [glycogenosis] (71,72) | |
Dent disease (73) |
Brackets contain potentially useful additional tests to further investigate if suspected. SPE, serum protein electrophoresis; UPE, urine protein electrophoresis; SFLCs, serum free light chains; WBCs, white blood cells; Gal-1-PUT, galactose-1-phosphate uridyl transferase; RBCs, red blood cells.
Tumour induced osteomalacia (TIO)
TIO—also known as oncogenic osteomalacia—is a rare syndrome characterised by the overproduction of FGF-23 usually (but not exclusively) by phosphaturic mesenchymal tumours (PMTs) (64). Excess FGF-23 leads to decreased maximum TRP, which in turn leads to renal phosphate wasting and hypophosphataemia (64). Over time, this phosphate wasting leads to osteomalacia/rickets due to decreased bone mineralisation, and decreased muscle function which can cause myopathy (64).
TIO is notoriously difficult to accurately diagnose, and time from symptom onset to diagnosis can be as high as 28 years (64). This is due to two main reasons. TIO is largely a diagnosis of exclusion, and the FGF-23 secreting PMTs can be difficult to locate as they can be found anywhere in the body (64). Symptoms of TIO include musculoskeletal pain, muscle weakness and bone insufficiency fractures, which are usually a consequence of hypophosphataemia rather than the frequently small and slow growing PMTs (64).
Laboratory tests and results useful to help to diagnose TIO include low TmP/GFR {section “Urine phosphate measurement—tubular maximum reabsorption [tubular maximum reabsorption rate of phosphate to glomerular filtration rate (TmP/GFR)]”}, high blood FGF-23, high blood alkaline phosphatase (ALP), normal blood calcium, normal blood PTH and low blood 1,25-dihydroxyvitamin D (which can in turn sometimes cause a high PTH via secondary mechanisms). Although FGF-23 analysis can be of benefit in suspected cases, FGF-23 is currently not easily available in many parts of the world due to difficulty with accurate quantification and poor assay standardisation, therefore the majority of available immunoassays are for research use only. In practice, TIO can be diagnosed without FGF-23 analysis when other causes of hypophosphataemia—including vitamin D deficiency, malabsorption, hyperparathyroidism, medications, poor diet, Fanconi syndrome, hereditary forms of FGF-23 dependent and independent causes of renal phosphate wasting, McCune-Albright syndrome etc.—have been excluded, but locating a potential PMT can still be difficult (64). The location of a potential PMT makes the diagnosis more likely, but other causes of hypophosphataemia should still be excluded as multiple factors can be present at the same time. The diagnosis can often only be confirmed when normophosphataemia occurs after the PMT is removed/destroyed. Due to the difficulty of diagnosing and treating TIO, suspected cases should be referred to specialist centres for diagnostic work up and management.
Post-renal transplant
Hypophosphataemia presents in 40% to 90% of patients one month after successful kidney transplant (65). The exact mechanism is not completely understood and is debated, but is likely due to a combination of tertiary hyperparathyroidism and chronically elevated FGF-23 leading to continued renal phosphate wasting post-transplant, as well as the increased incidence of vitamin D deficiency associated with this population group (65,66). Interestingly, factors associated with a good response to the transplant—including pre-transplant hyperparathyroidism, living kidney donor, lower donor age, etc.—are associated with an increased incidence of post-renal transplant hypophosphataemia (66).
Vitamin D dependent rickets (VDDR)
VDDR can be complex to diagnose and manage due to many inherited and acquired causes, therefore suspected cases should be referred to specialist centres. Hypophosphataemia is the causative abnormality in all forms of rickets (67). Both calcipaenic and phosphopaenic rickets result in renal phosphate wasting and hypophosphataemia. As phosphate is required for the normal apoptosis of hypertrophic chondrocytes (68), the resulting hypophosphataemia prevents growth plate maturation and directly causes the clinical features associated with rickets. See Levine (67) for an open access overview of VDDR.
Other causes of acquired increased urinary phosphate excretion (phosphate wasting)
Other (non-exhaustive) causes of acquired renal phosphate wasting leading to hypophosphataemia include hepatic resection (69) and severe burns requiring high volume haemofiltration (70).
Low urinary phosphate excretion (normal/high TmP-GFR)
Low urinary phosphate excretion (normal/high TmP-GFR) in the presence of hypophosphataemia indicates that there is appropriate renal reabsorption of phosphate, suggesting that the primary abnormality is not the kidneys and is more likely to be vitamin D deficiency, poor intake, malabsorption or another cause outlined in Figure 4.
Vitamin D deficiency
If calcium is also low, vitamin D deficiency should be considered by assessing risk factors—including poor diet, poor sunlight exposure, high skin surface area coverage, etc.—in the presence of other relevant presentations including bone pain, bone malformation, high PTH, high ALP etc. Although testing for 25-hydroxyvitamin D is available, routinely available immunoassays have limitations. For example, 25-hydroxyvitamin D assays tend to measure the circulating concentration of total vitamin D, which includes the majority (~85%) of vitamin D that is bound to vitamin D binding protein (VDBP) (71). The ratio of VDBP-25-OH vitamin D complexes to free 25-OH vitamin D affects the overall biological activity of circulating vitamin D (72), therefore the total circulating concentrations may not reflect the true physiological status. This is particularly true in patients who have chronic inflammation (73), pregnant or are taking hormonal replacement therapy (74,75). In reality, testing for 25-OH vitamin D is not required to confirm deficiency as normalisation of biochemical abnormalities, and alleviation of relevant symptoms, after vitamin D replacement more accurately confirms vitamin D deficiency as the primary cause.
Poor phosphate intake and malabsorption
Although rare, poor dietary phosphate intake should be considered in some cases, particularly in patients with anorexia nervosa and chronically high alcohol intake. Malabsorption can also cause hypophosphataemia and can be caused by many different conditions including inflammatory bowel disease, chronic diarrhoea, cystic fibrosis, pancreatic insufficiency, short bowel syndrome, etc. (76). Low dietary intake of phosphate only is likely a rare cause of hypophosphataemia (77), and in extreme cases of malnutrition, hypophosphataemia is usually combined with multiple deficiencies including vitamin D, exacerbating the presentation and biochemistry. Parenteral nutrition may exacerbate hypophosphataemia if not adequately balanced (78). There is no gold standard test to diagnose poor dietary intake and malabsorption, therefore investigation of suspected cases needs to be guided by specific patient presentations and other detectable abnormalities (76). The work up of suspected malabsorption will not be discussed here as it has been comprehensively covered elsewhere (76).
Transcellular shift of phosphate
Many situations can lead to extracellular phosphate moving into the intracellular compartment, leading to acute hypophosphataemia. Panic attacks, which can present in patients who are particularly needle phobic, can result in an acute respiratory alkalosis that can cause non-clinically significant hypophosphataemia (49). Metabolic alkalosis can also lead to hypophosphataemia by a similar mechanism of increasing the activity of phosphofructokinase, resulting in increased intracellular phosphate demand (79). RFS can result upon refeeding, both enterally and parenterally, after prolonged periods of malnutrition. There is no official defining feature of RFS, but the majority of RFS studies use a low or decreasing phosphate as part of the diagnostic criteria (80), and hypokalaemia, hypomagnesaemia and low blood thiamine (vitamin B1) are also commonly present. Patients with a very low body mass index (<16 kg/m2), and patients who have had little or no nutritional intake for >10 days, are at particularly increased risk of developing RFS upon refeeding (81). Therefore, care must be taken when refeeding patients at high risk, and national and local refeeding guidance should be followed. Some of the drugs outlined in Table 2 can also cause acute shifts of phosphate into cells.
As the name suggests, hungry bone syndrome (HBS) refers to translocation of minerals to bone, usually after a definitive treatment such as parathyroidectomy for hyperparathyroidism (82). A reduction in serum phosphate, calcium and magnesium is frequently seen. HBS is unlikely to cause diagnostic confusion because it is only seen after significant procedures, but is still important to identify and correct to avoid long term morbidity.
Genomic causes
The genomic causes of hypophosphataemia are numerous and can be very complex to diagnose. These will not be discussed in detail here as suspected cases should be referred to specialist centres for work up and management. For context and potential diagnostic direction, Table 5 outlines some of the genetic causes of hypo- and hyperphosphataemia (2,86-90).
Table 5
Condition name | Genetics and/or mechanism | Low TmP/GFR | FGF-23 | 1,25 Vit D | PTH |
---|---|---|---|---|---|
Hypophosphataemia | |||||
Hereditary hypophosphataemic rickets with hypercalciuria (HHRH) | Autosomal recessive, loss of function of NPT2c (heterozygotes can have isolated hypercalciuria) | Present | Low | High | Low/normal |
Fanconi syndrome | Damage of proximal tubule secondary to many different inherited conditions e.g., cystinosis, tyrosinaemia | Present | Low | Low/normal | Low/normal |
X-linked hypophosphataemia (XLH)a | X-linked, loss of function of PHEX (which cleaves and thus inactivates FGF-23) | Present | High | Low/normal | Normal/high |
Autosomal dominant hypophosphataemic rickets (ADHR) | Autosomal dominant, gain of function FGF-23 (inactivating cleavage site is mutated, maintaining activity) | Present | Highb | Low/normal | Normal/high |
Autosomal recessive hypophosphataemic rickets (ARHR) | Autosomal recessive, loss of function of DMP1 |
Present | High | Low/normal | Normal/high |
Hypophosphataemic rickets and hyperparathyroidism | Gain of function in α-klotho | Present | High | Normal/high | High |
Jansen’s metaphyseal chondrodysplasia | Activating mutation of the PTH receptor (PTHR1) | Present | High | Normal | Low/Normal |
Polyostotic fibrous dysplasia associated with McCune-Albright syndrome | GNAS1, abnormal osteogenic cells can release FGF-23 in approximately 50% | Present | High | Low/normal | Normal/high |
Autosomal recessive hypophosphataemic rickets type 2 (ARHR2) | Autosomal recessive, loss of function causing deficiency of ENPP1 resulting in increased FGF-23 | Present | High | Normal | Normal/high |
Raine syndrome | Autosomal recessive, loss of function FAM20C, kinase that acts on FGF-23 and DMP1 among others | Present | High | High | High |
Hyperphosphataemia | |||||
Hyperphosphataemic familial tumoural calcinosis (HFTC)c (83) | Autosomal recessive, loss of function of FGF-23, unstable intact protein | Absent | Low/normald | Normal/high | Normal |
Autosomal recessive, loss of function of GALNT3e, glycosylation defects lead to proteolysis and hence reduction of FGF-23 | Absent | Low/normald | Normal/high | Normal | |
Autosomal recessive, loss of function in α-klotho | Absent | High (very) | High | High |
a, previously/also known as adult-onset vitamin D resistant hypophosphataemic rickets. b, disease phenotype associated with FGF-23 concentration. c, there is an acquired autoimmune variant of this condition associated with antibodies to FGF-23 (83,84). d, in tumoural calcinosis, all conditions have high concentrations of C-terminal fragments of FGF-23, but concentration of intact FGF-23 varies (see table). Therefore, the results will depend on whether the assay measures both intact and C-terminal FGF-23 or intact only (85). e, hyperostosis-hyperphosphataemia syndrome (HHS) was found to be a clinical phenotype of tumoural calcinosi caused by identical mutations in GALNT3. TmP/GFR, tubular maximum reabsorption rate of phosphate to glomerular filtration rate; FGF-23, fibroblast growth factor 23; 1,25 Vit D, 1,25-dihydroxyvitamin D; PTH, parathyroid hormone; DMP1, dentin matrix acid phosphprotein 1; ENPP1, ectonucleotide pyrophosphatase/phosphodiesterase-1.
HyperphosphataemiaOther Section
- Introduction
- Methods
- Background
- Hypophosphataemia
- Hyperphosphataemia
- Special states
- Conclusions
- Acknowledgments
- Footnote
- References
Hyperphosphataemia can be defined as a blood phosphate concentration >1.45 mmol/L (>5.00 mg/dL) (8). Severe hyperphosphataemia can be loosely defined as a blood phosphate concentration >2.00 mmol/L (>6.20 mg/dL) (8), although factors including the speed of increase and other relevant conditions will affect the clinical urgency. Hyperphosphataemia has a wide prevalence of between 5.6% to 67.9% in critically ill patients depending on the cause of illness (91). The prevalence in end stage renal failure (ESRF) is between 50% and 74% (92). Symptoms are usually mild when chronic, but can cause symptoms of hypocalcaemia—including seizures and tetany—when acute due to calcium phosphate salt deposition in tissues (92).
There are three main categories of situations that can cause hyperphosphataemia. These include large phosphate load, inappropriate increased renal reabsorption, or renal failure (93). As with the majority of laboratory tests, false elevations should also always be considered if the hyperphosphataemia is unexpected and does not fit the clinical picture in order to avoid unnecessary (and possibly dangerous) investigation and treatment. A proposed algorithm for the laboratory investigation of hyperphosphataemia is shown in Figure 5.
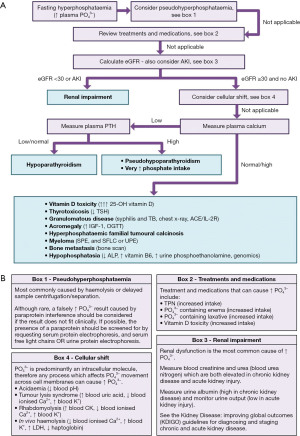
Pseudohyperphosphataemia
As phosphate is 100× more concentrated in red blood cells (RBCs) than in extracellular fluid (including the blood), any in vitro process which disturbs RBCs, or alters their metabolism, can lead to spurious hyperphosphataemia. For example, in vitro haemolysis causes the release of intracellular phosphate into the blood and can cause a falsely high result (94), but this is usually easily identified by laboratories due to the red colour of free haemoglobin in plasma. Delayed sample centrifugation and separation of the plasma from the RBCs (4–6 hours) will cause intracellular phosphate to leak into the blood (5), but this is not a reflection of the in vivo phosphate status.
Although liposomal amphotericin B (antifungal) therapy and the presence of a paraprotein (e.g., myeloma, Waldenstrom macroglobulinaemia etc.) have been reported to cause pseudohypophosphataemia (see section “Pseudohypophosphataemia”), there are many more case reports of these causing pseudohyperphosphataemia (36,83-85,95-111). This suggests that these situations more commonly cause falsely high rather than falsely low phosphate results. Analysis using a different phosphate method, or removal of potentially interfering paraproteins from serum (112), can identify false elevations of phosphate results from these causes.
Heparin and alteplase therapy have been implicated in causing falsely high phosphate results (36). It is important that any dilutions of plasma in the laboratory are done with appropriate phosphate free media to avoid overestimating the in vivo concentration (113). Endogenous high concentrations of lipids and bilirubin have additionally been reported to cause pseudohyperphosphataemia (36), but similarly to haemolysis, laboratories should not release false results from these mechanisms as these interferences are detectable.
Treatments and medications
Once pseudohyperphosphataemia has been excluded, the next step should be to review if any recent treatments or medications may be the explanation. Patients on total parenteral nutrition (TPN) (114), or intravenous replacement (115), should have their phosphate intake assessed as this may need to be reduced if there is a low risk of RFS, particularly if there is concurrent CKD or acute kidney injury (AKI). Enemas and laxatives containing high concentrations of phosphate can cause acute and dangerous hyperphosphataemia (116,117), although newer formulations are less of a risk (118). Exogenous vitamin D toxicity can cause hyperphosphataemia due its effect of increasing intestinal absorption. However, this is rare in practice due to renal 1α-hydroxylation regulation, and plasma 25-OH vitamin D levels typically need to exceed 375 nmol/L (150 ng/mL) for acute toxicity or be 125–375 nmol/L (50–150 ng/mL) for chronic toxicity (119). The diagnosis of vitamin D toxicity should be clinically led in the presence of relevant symptoms and history, and extra consideration should be given to patients who are taking high doses of supplements and have a granulomatous disease (sarcoidosis, lymphoma, tuberculosis) that can independently convert the 25-OH vitamin D to active 1,25-dihydroxyvitamin D (119).
Renal failure
Hyperphosphataemia is a well described phenomenon in chronic and acute renal failure and adversely affects outcome (120,121). However, hyperphosphataemia in chronic renal failure is not a reliable finding, and differences in a population’s phosphate intake—e.g., in carbonated beverages—and food supplementation with vitamin D, may all affect the likelihood of developing the biochemical disturbance (122). If suspected, renal function should be assessed by measuring blood creatinine (with or without blood urea), and this is usually done at the same time as blood phosphate assessment. The estimated glomerular filtration rate (eGFR) should be calculated to grade CKD, and an eGFR <30 mL/min/1.73 m2 typically leads to hyperphosphataemia (although this can vary depending on other factors) (123). AKI—which can be present with an eGFR >60 mL/min and is defined by an acute change in blood creatinine—can also be a renal failure cause of hyperphosphataemia and should always be ruled out. See the Kidney Disease: Improving Global Outcomes 2012 guidelines (124) for information on the diagnosis and management of AKI.
Cellular shift
Causes of phosphate shift from the intracellular compartment to blood—including tumour lysis syndrome, rhabdomyolysis, acidaemia and in vivo haemolysis—also frequently cause hyperkalaemia. See the hyperkalaemia section in the open access article on potassium in the previous series in the journal (125) for more information.
Calcium and PTH analysis
As a general guide during hyperphosphataemia, calcium is usually low when PTH activity is inappropriately low. If the measured PTH is inappropriately low/normal during concurrent hypocalcaemia, this is consistent with hypoparathyroidism [see calcium article within this series for more information (6)]. If the measured PTH is high during hypocalcaemia, this suggests that PTH is being appropriately secreted but it is not having the appropriate end organ effects to increase calcium and decrease phosphate. This may be consistent with pseudohypoparathyroidism which is a rare end organ resistance to the effects of PTH, and can cause a very similar biochemical pattern to hypoparathyroidism with the exception of a high plasma PTH (126). If suspected, GNAS mutations analysis may be helpful, but this is not always required for a diagnosis (126) [see Linglart et al. (126) for more information]. Very high levels of phosphate intake may also cause hyperphosphataemia and a high PTH in attempt to increase excretion of the excess, and hypocalcaemia as the excess phosphate forms calcium phosphate salts that are deposited in tissues (5).
Other (including hypophosphatasia)
Other, less common, causes of hyperphosphataemia are outlined in the final box in the flowchart in Figure 5A. The majority of these are very rare causes of hyperphosphataemia and more commonly cause hypercalcaemia, therefore the reader is directed to the calcium article within this series for further information (6). A particularly phosphate relevant diagnosis is hypophosphatasia, which is a very rare genomic condition affecting the activity of tissue non-specific ALP (127). This can cause issues with bone and teeth mineralisation, and can be diagnosed in child or adulthood (127). The main biochemical feature of hypophosphatasia is a persistently lower ALP activity than expected for age, but hypophosphataemia is also frequently present (127). High blood pyridoxal 5’-phosphate (major circulating form of vitamin B6) (128), and high urinary excretion of phosphoethanolamine (urine amino acid analysis) (129), are consistent with (but not pathognomonic) for hypophosphatasia, particularly in the presence of low ALP activity and relevant bone/teeth presentations.
Genomic causes
Inheritable hyperphosphataemia—called hyperphosphataemic familial tumoural calcinosis (HFTC)—is primarily driven by mutations reducing FGF-23 concentration or inactivation of the receptors (see Table 5). Similarly to hypophosphataemia, genomic causes are complex to diagnose and should be referred to specialist centres for work up and management, therefore they will not be discussed in detail here.
Special statesOther Section
- Introduction
- Methods
- Background
- Hypophosphataemia
- Hyperphosphataemia
- Special states
- Conclusions
- Acknowledgments
- Footnote
- References
Paediatric
Phosphate concentrations are higher in children and reduce as they get older (Table 6) until they reach adulthood. As phosphate requirements in infants is high due to rapid bone formation, phosphate deficiency can occur in breast fed infants if the concentration of phosphate in human breast milk is low. Consequently, there is a higher risk if the mother is hypophosphataemic before and during breast feeding (131).
Table 6
Age | Lower limit | Upper limit | Sample size | Lower confidence limits | Upper confidence limits |
---|---|---|---|---|---|
0 to <15 days | 1.8 | 3.4 | 408 | (1.73, 1.90) | (3.29, 3.47) |
15 days to <1 year | 1.54 | 2.72 | 288 | (1.35, 1.63) | (2.62, 2.79) |
1 to <5 years | 1.38 | 2.19 | 368 | (1.29, 1.45) | (2.11, 2.39) |
5 to <13 years | 1.33 | 1.92 | 704 | (1.31, 1.35) | (1.89, 1.95) |
13 to <16 years | |||||
Female | 1.02 | 1.79 | 95 | (0.98, 1.06) | (1.73, 1.84) |
Male | 1.14 | 1.99 | 95 | (1.10, 1.17) | (1.93, 2.04) |
16 to <19 years | 0.95 | 1.62 | 374 | (0.87, 1.01) | (1.58, 1.82) |
Based on a CALIPER study (130) of healthy children and adolescents (newborn to 18 years of age) from a multiethnic population and measured using the Abbott Architect analyser.
Pregnancy and lactation
In a limited study in Africa, where various serum measurements were taken, phosphate concentrations in pregnancy did not appear to change during various gestational stages (132,133). An earlier study did not describe any phosphate concentration changes during pregnancy, but did note an increase in phosphate concentration during lactation. A paper with the primary aim of assessing caffeine’s effect on vitamin D levels in pregnancy demonstrated that phosphate levels are within the reference interval during pregnancy (134).
ConclusionsOther Section
- Introduction
- Methods
- Background
- Hypophosphataemia
- Hyperphosphataemia
- Special states
- Conclusions
- Acknowledgments
- Footnote
- References
This article presents a laboratory approach to help diagnose the principal causes of phosphate disturbances after the exclusion of commonly encountered false results. These algorithms provide a practical approach in daily clinical practice to help guide investigations and elucidate the reasons for the biochemical derangement, but it is not a substitute for clinical assessment and expert guidelines; rare genomic causes and abnormalities in children should be referred to expert centres.
The strength of this review is that it is based on established practice and aimed at being applicable for most health care settings, focussed on common presentations, and accessible for all users. The limitations are that a critical appraisal or systematic review were outside the scope of the article, and also that some special patient groups may not be well represented, e.g., neonatal. The cost and clinical efficiency of such proposed algorithms are therefore unknown, but by providing the suggested approach, this will allow pathways to be validated and the performance quantified if implemented. We hope to provide a framework for clinicians to consider an approach to further diagnostics when facing clinical conundrums related to abnormal phosphate results.
AcknowledgmentsOther Section
- Introduction
- Methods
- Background
- Hypophosphataemia
- Hyperphosphataemia
- Special states
- Conclusions
- Acknowledgments
- Footnote
- References
Funding: None.
FootnoteOther Section
- Introduction
- Methods
- Background
- Hypophosphataemia
- Hyperphosphataemia
- Special states
- Conclusions
- Acknowledgments
- Footnote
- References
Provenance and Peer Review: This article was commissioned by the editorial office, Journal of Laboratory and Precision Medicine for the series “Investigative Algorithms in Laboratory Medicine II: Focus on Bone and Liver”. The article has undergone external peer review.
Reporting Checklist: The authors have completed the Narrative Review reporting checklist. Available at https://jlpm.amegroups.org/article/view/10.21037/jlpm-24-35/rc
Peer Review File: Available at https://jlpm.amegroups.org/article/view/10.21037/jlpm-24-35/prf
Conflicts of Interest: All authors have completed the ICMJE uniform disclosure form (available at https://jlpm.amegroups.org/article/view/10.21037/jlpm-24-35/coif). The series “Investigative Algorithms in Laboratory Medicine II: Focus on Bone and Liver” was commissioned by the editorial office without any funding or sponsorship. K.E.S. served as the unpaid Guest Editor of the series and serves as the editorial board member of the Journal of Laboratory and Precision Medicine from September 2022 to August 2024. N.J.L.G. declares consulting fees from Takeda Pharma, Ascendis Pharma, and UCB Pharma, and serves as Chair for the Royal Osteoporosis Society and the Specialised Endocrinology Clinical Reference Group for National Health Service England Improvement, and as Global Co-Chair for the PARADIGHM patient registry for hypoparathyroidism (Takeda supported). The authors have no other conflicts of interest to declare.
Ethical Statement: The authors are accountable for all aspects of the work in ensuring that questions related to the accuracy or integrity of any part of the work are appropriately investigated and resolved.
Open Access Statement: This is an Open Access article distributed in accordance with the Creative Commons Attribution-NonCommercial-NoDerivs 4.0 International License (CC BY-NC-ND 4.0), which permits the non-commercial replication and distribution of the article with the strict proviso that no changes or edits are made and the original work is properly cited (including links to both the formal publication through the relevant DOI and the license). See: https://creativecommons.org/licenses/by-nc-nd/4.0/.
ReferencesOther Section
- Introduction
- Methods
- Background
- Hypophosphataemia
- Hyperphosphataemia
- Special states
- Conclusions
- Acknowledgments
- Footnote
- References
- Goretti Penido M, Alon US. Phosphate homeostasis and its role in bone health. Pediatr Nephrol 2012;27:2039-48. [Crossref] [PubMed]
- Farrow EG, White KE. Recent advances in renal phosphate handling. Nat Rev Nephrol 2010;6:207-17. [Crossref] [PubMed]
- Berndt T, Kumar R. Phosphatonins and the regulation of phosphate homeostasis. Annu Rev Physiol 2007;69:341-59. [Crossref] [PubMed]
- Iheagwara OS, Ing TS, Kjellstrand CM, et al. Phosphorus, phosphorous, and phosphate. Hemodial Int 2013;17:479-82. [Crossref] [PubMed]
- Manghat P, Sodi R, Swaminathan R. Phosphate homeostasis and disorders. Ann Clin Biochem 2014;51:631-56. [Crossref] [PubMed]
- Flowers KC, Shipman KE, Shipman AR, et al. Investigative algorithms for disorders causing hypercalcaemia and hypocalcaemia: a narrative review. J Lab Precis Med 2024;9:27. [Crossref]
- Bollerslev J, Rejnmark L, Zahn A, et al. European Expert Consensus on Practical Management of Specific Aspects of Parathyroid Disorders in Adults and in Pregnancy: Recommendations of the ESE Educational Program of Parathyroid Disorders. Eur J Endocrinol 2022;186:R33-63. [Crossref] [PubMed]
- Koumakis E, Cormier C, Roux C, et al. The Causes of Hypo- and Hyperphosphatemia in Humans. Calcif Tissue Int 2021;108:41-73. [Crossref] [PubMed]
- Noori N, Sims JJ, Kopple JD, et al. Organic and inorganic dietary phosphorus and its management in chronic kidney disease. Iran J Kidney Dis 2010;4:89-100. [PubMed]
- Bartter FC. Reporting of phosphate and phosphorus plasma values. Am J Med 1981;71:848. [Crossref] [PubMed]
- Christov M, Jüppner H. Phosphate homeostasis disorders. Best Pract Res Clin Endocrinol Metab 2018;32:685-706. [Crossref] [PubMed]
- Levi M, Gratton E, Forster IC, et al. Mechanisms of phosphate transport. Nat Rev Nephrol 2019;15:482-500. [Crossref] [PubMed]
- Nam HK, Liu J, Li Y, et al. Ectonucleotide pyrophosphatase/phosphodiesterase-1 (ENPP1) protein regulates osteoblast differentiation. J Biol Chem 2011;286:39059-71. [Crossref] [PubMed]
- Khundmiri SJ, Murray RD, Lederer E. PTH and Vitamin D. Compr Physiol 2016;6:561-601. [Crossref] [PubMed]
- Smit MA, van Kinschot CMJ, van der Linden J, et al. Clinical Guidelines and PTH Measurement: Does Assay Generation Matter? Endocr Rev 2019;40:1468-80. [Crossref] [PubMed]
- Navarro‐García JA, Fernández‐Velasco M, Delgado C, et al. PTH, vitamin D, and the FGF‐23–klotho axis and heart: Going beyond the confines of nephrology. Eur J Clin Invest 2018;48:e12902. [Crossref] [PubMed]
- Quarles LD. Fibroblast growth factor 23 and α-Klotho co-dependent and independent functions. Curr Opin Nephrol Hypertens 2019;28:16-25. [Crossref] [PubMed]
- Guo YC, Yuan Q. Fibroblast growth factor 23 and bone mineralisation. Int J Oral Sci 2015;7:8-13. [Crossref] [PubMed]
- Donovan K, Herrington WG, Paré G, et al. Fibroblast Growth Factor-23 and Risk of Cardiovascular Diseases: A Mendelian Randomization Study. Clin J Am Soc Nephrol 2023;18:17-27. [Crossref] [PubMed]
- Ovejero D, Hartley IR, de Castro Diaz LF, et al. PTH and FGF23 Exert Interdependent Effects on Renal Phosphate Handling: Evidence From Patients With Hypoparathyroidism and Hyperphosphatemic Familial Tumoral Calcinosis Treated With Synthetic Human PTH 1-34. J Bone Miner Res 2022;37:179-84. [Crossref] [PubMed]
- Ackah SA, Imel EA. Approach to Hypophosphatemic Rickets. J Clin Endocrinol Metab 2022;108:209-20. [Crossref] [PubMed]
- Murer H, Hernando N, Forster I, et al. Proximal tubular phosphate reabsorption: molecular mechanisms. Physiol Rev 2000;80:1373-409. [Crossref] [PubMed]
- Becker GJ, Walker RG, Hewitson TD, et al. Phosphate levels—time for a rethink? Nephrol Dial Transplant 2009;24:2321-4. [Crossref] [PubMed]
- Trivedi H, Szabo A, Zhao S, et al. Circadian variation of mineral and bone parameters in end-stage renal disease. J Nephrol 2015;28:351-9. [Crossref] [PubMed]
- Fraser WD, Logue FC, Christie JP, et al. Alteration of the circadian rhythm of intact parathyroid hormone following a 96-hour fast. Clin Endocrinol (Oxf) 1994;40:523-8. [Crossref] [PubMed]
- Bajaña W, Aranda E, Arredondo ME, et al. Impact of an Andean breakfast on biochemistry and immunochemistry laboratory tests: an evaluation on behalf COLABIOCLI WG-PRE-LATAM. Biochem Med (Zagreb) 2019;29:020702. [Crossref] [PubMed]
- Lima-Oliveira G, Salvagno GL, Lippi G, et al. Influence of a regular, standardized meal on clinical chemistry analytes. Ann Lab Med 2012;32:250-6. [Crossref] [PubMed]
- Hoffmann M, Zemlin AE, Meyer WP, et al. Hypophosphataemia at a large academic hospital in South Africa. J Clin Pathol 2008;61:1104-7. [Crossref] [PubMed]
- Al Harbi SA, Al-Dorzi HM, Al Meshari AM, et al. Association between phosphate disturbances and mortality among critically ill patients with sepsis or septic shock. BMC Pharmacol Toxicol 2021;22:30. [Crossref] [PubMed]
- Hadavi M, Taghinezhad F, Shafiei E, et al. Hypo- and Hyperphosphatemia at Admission as Independent Factors of Mortality of COVID-19 Patients: Findings from a Retrospective Cohort Study. Int J Endocrinol Metab 2022;20:e126386. [Crossref] [PubMed]
- Bsar AEME, El-Wakiel SA, El-Harrisi MA, et al. Frequency and Risk Factors of Hypophosphatemia in Patients Admitted to Emergency Intensive Care Unit in Zagazig University Hospitals. Indian J Crit Care Med 2023;27:277-82. [Crossref] [PubMed]
- Adhikari S, Mamlouk O, Rondon-Berrios H, et al. Hypophosphatemia in cancer patients. Clin Kidney J 2021;14:2304-15. [Crossref] [PubMed]
- Kagansky N, Levy S, Koren-Morag N, et al. Hypophosphataemia in old patients is associated with the refeeding syndrome and reduced survival. J Intern Med 2005;257:461-8. [Crossref] [PubMed]
- Statlender L, Raphaeli O, Bendavid I, et al. Correlations between First 72 h Hypophosphatemia, Energy Deficit, Length of Ventilation, and Mortality-A Retrospective Cohort Study. Nutrients 2022;14:1332. [Crossref] [PubMed]
- Reintam Blaser A, Gunst J, Ichai C, et al. Hypophosphatemia in critically ill adults and children - A systematic review. Clin Nutr 2021;40:1744-54. [Crossref] [PubMed]
- Molinaris V, Bianchetti MG, Milani GP, et al. Interferences in the measurement of circulating phosphate: a literature review. Clin Chem Lab Med 2020;58:1971-7. [Crossref] [PubMed]
- Dimeski G, Hamer A, Cooper C, et al. Pseudohypophosphataemia secondary to paraproteinaemia may occur without the presence of hypergammaglobulinaemia. Pathology 2016;48:102-3. [Crossref] [PubMed]
- Wen Wu L, Choi TS, Barbosa M, et al. PSEUDOHYPOPHOSPHATEMIA IN A PATIENT WITH MULTIPLE MYELOMA. AACE Clin Case Rep 2020;6:e334-7. [Crossref] [PubMed]
- Mao Z, Ong AC. Spurious hypophosphatemia associated with monoclonal paraproteinemia. QJM 2012;105:693-6. [Crossref] [PubMed]
- Rajkumar SV, Dimopoulos MA, Palumbo A, et al. International Myeloma Working Group updated criteria for the diagnosis of multiple myeloma. Lancet Oncol 2014;15:e538-48. [Crossref] [PubMed]
- Flowers KC, Tuddenham E, Leiva A, et al. Negative interference from immunoglobulin M paraproteinaemia on the Roche enzymatic creatinine method. Ann Clin Biochem 2022;59:205-10. [Crossref] [PubMed]
- Polak R, Huisman A, Sikma MA, et al. Spurious hypokalaemia and hypophosphataemia due to extreme hyperleukocytosis in a patient with a haematological malignancy. Ann Clin Biochem 2010;47:179-81. [Crossref] [PubMed]
- Duncanson GO, Worth HG. Pseudohypophosphataemia as a result of bilirubin interference. Ann Clin Biochem 1990;27:253-7. [Crossref] [PubMed]
- Donhowe JM, Freier EF, Wong ET, et al. Factitious hypophosphatemia related to mannitol therapy. Clin Chem 1981;27:1765-9. [Crossref] [PubMed]
- McCoy MT, Aguanno JJ, Ritzmann SE. Interferences of mannitol with phosphate determination. Am J Clin Pathol 1982;77:468-70. [Crossref] [PubMed]
- Eisenbrey AB, Mathew R, Kiechle FL. Mannitol interference in an automated serum phosphate assay. Clin Chem 1987;33:2308-9. [Crossref] [PubMed]
- Megapanou E, Florentin M, Milionis H, et al. Drug-Induced Hypophosphatemia: Current Insights. Drug Saf 2020;43:197-210. [Crossref] [PubMed]
- Mays JA, Greene DN, Poon A, et al. Pseudohypophosphatemia associated with high-dose liposomal amphotericin B therapy. Clin Biochem 2017;50:967-71. [Crossref] [PubMed]
- Datta BN, Stone MD. Hyperventilation and hypophosphataemia. Ann Clin Biochem 2009;46:170-171. [Crossref] [PubMed]
- Insogna KL, Bordley DR, Caro JF, et al. Osteomalacia and weakness from excessive antacid ingestion. JAMA 1980;244:2544-6. [Crossref] [PubMed]
- Gonzalez Ballesteros LF, Ma NS, Gordon RJ, et al. Unexpected widespread hypophosphatemia and bone disease associated with elemental formula use in infants and children. Bone 2017;97:287-92. [Crossref] [PubMed]
- Seitz DP. Hypophosphatemia and neuroleptic malignant syndrome. J Clin Psychopharmacol 2007;27:302-3. [Crossref] [PubMed]
- Martens KL, Wolf M. Incidence, mechanism, and consequences of IV iron-induced hypophosphatemia. Hematology Am Soc Hematol Educ Program 2023;2023:636-9. [Crossref] [PubMed]
- Schaefer B, Tobiasch M, Wagner S, et al. Hypophosphatemia after intravenous iron therapy: Comprehensive review of clinical findings and recommendations for management. Bone 2022;154:116202. [Crossref] [PubMed]
- Vilaca T, Velmurugan N, Smith C, et al. Osteomalacia as a Complication of Intravenous Iron Infusion: A Systematic Review of Case Reports. J Bone Miner Res 2022;37:1188-99. [Crossref] [PubMed]
- Bishay RH, Ganda K, Seibel MJ. Long-term iron polymaltose infusions associated with hypophosphataemic osteomalacia: a report of two cases and review of the literature. Ther Adv Endocrinol Metab 2017;8:14-9. [Crossref] [PubMed]
- Samões B, Silva B, Martins A, et al. Hypophosphatemic osteomalacia induced by intravenous iron therapy: a case report. Joint Bone Spine 2023;90:105586. [Crossref] [PubMed]
- Bagnis CI, Karie S, Deray G, et al. Hypophosphataemia: an easy strategy for diagnosis and treatment in HIV patients. Antivir Ther 2009;14:481-8. [Crossref] [PubMed]
- Payne RB. Renal tubular reabsorption of phosphate (TmP/GFR): indications and interpretation. Ann Clin Biochem 1998;35:201-6. [Crossref] [PubMed]
- Choi HS, Kwon A, Chae HW, et al. Respiratory failure in a diabetic ketoacidosis patient with severe hypophosphatemia. Ann Pediatr Endocrinol Metab 2018;23:103-6. [Crossref] [PubMed]
- Centeno PP, Herberger A, Mun HC, et al. Phosphate acts directly on the calcium-sensing receptor to stimulate parathyroid hormone secretion. Nat Commun 2019;10:4693. [Crossref] [PubMed]
- Lecoq AL, Chaumet-Riffaud P, Blanchard A, et al. Hyperparathyroidism in Patients With X-Linked Hypophosphatemia. J Bone Miner Res 2020;35:1263-73. [Crossref] [PubMed]
- Foreman JW. Fanconi Syndrome. Pediatr Clin North Am 2019;66:159-67. [Crossref] [PubMed]
- Minisola S, Peacock M, Fukumoto S, et al. Tumour-induced osteomalacia. Nat Rev Dis Primers 2017;3:17044. [Crossref] [PubMed]
- Ghorbani M, Ossareh S. Early postkidney transplantation hypophosphatemia. J Res Med Sci 2020;25:36. [Crossref] [PubMed]
- Ralston MR, Stevenson KS, Mark PB, et al. Clinical factors associated with severe hypophosphataemia after kidney transplant. BMC Nephrol 2021;22:407. [Crossref] [PubMed]
- Levine MA. Diagnosis and Management of Vitamin D Dependent Rickets. Front Pediatr 2020;8:315. [Crossref] [PubMed]
- Sabbagh Y, Carpenter TO, Demay MB. Hypophosphatemia leads to rickets by impairing caspase-mediated apoptosis of hypertrophic chondrocytes. Proc Natl Acad Sci U S A 2005;102:9637-42. [Crossref] [PubMed]
- Salem RR, Tray K. Hepatic resection-related hypophosphatemia is of renal origin as manifested by isolated hyperphosphaturia. Ann Surg 2005;241:343-8. [Crossref] [PubMed]
- Kail D, Arif F, Sultan-Ali I, et al. A Retrospective Chart Review to Determine Hypophosphatemia Incidence and Phosphorus Supplementation Requirements in Patients With Severe Thermal Cutaneous Injuries Receiving High-Volume Hemofiltration. J Burn Care Res 2023;44:234-9. [Crossref] [PubMed]
- Delrue C, Speeckaert MM. Vitamin D and Vitamin D-Binding Protein in Health and Disease. Int J Mol Sci 2023;24:4642. [Crossref] [PubMed]
- Bikle DD, Schwartz J, Vitamin D. Binding Protein, Total and Free Vitamin D Levels in Different Physiological and Pathophysiological Conditions. Front Endocrinol (Lausanne) 2019;10:317. [Crossref] [PubMed]
- Waldron JL, Ashby HL, Cornes MP, et al. Vitamin D: a negative acute phase reactant. J Clin Pathol 2013;66:620-2. [Crossref] [PubMed]
- Altieri B, Cavalier E, Bhattoa HP, et al. Vitamin D testing: advantages and limits of the current assays. Eur J Clin Nutr 2020;74:231-47. [Crossref] [PubMed]
- Fernando M, Ellery SJ, Marquina C, et al. Vitamin D-Binding Protein in Pregnancy and Reproductive Health. Nutrients 2020;12:1489. [Crossref] [PubMed]
- van der Heide F. Acquired causes of intestinal malabsorption. Best Pract Res Clin Gastroenterol 2016;30:213-24. [Crossref] [PubMed]
- Barron LJ, Barron RF, Johnson JCS, et al. A retrospective analysis of biochemical and haematological parameters in patients with eating disorders. J Eat Disord 2017;5:32. [Crossref] [PubMed]
- Imel EA, Econs MJ. Approach to the hypophosphatemic patient. J Clin Endocrinol Metab 2012;97:696-706. [Crossref] [PubMed]
- Allen GT, Shipman AR, Darragh-Hickey C, et al. Investigative algorithms for disorders affecting alkalosis: a narrative review. J Lab Precis Med 2022;7:23. [Crossref]
- Friedli N, Stanga Z, Sobotka L, et al. Revisiting the refeeding syndrome: Results of a systematic review. Nutrition 2017;35:151-60. [Crossref] [PubMed]
- Mehanna HM, Moledina J, Travis J. Refeeding syndrome: what it is, and how to prevent and treat it. BMJ 2008;336:1495-8. [Crossref] [PubMed]
- Witteveen JE, van Thiel S, Romijn JA, et al. Hungry bone syndrome: still a challenge in the post-operative management of primary hyperparathyroidism: a systematic review of the literature. Eur J Endocrinol 2013;168:R45-53. [Crossref] [PubMed]
- Knoderer CA, Knoderer HM. Hyperphosphatemia in pediatric oncology patients receiving liposomal amphotericin B. J Pediatr Pharmacol Ther 2011;16:87-91. [Crossref] [PubMed]
- Kritmetapak K, Dumrongsukit S, Jinchai J, et al. Pseudohyperphosphatemia in a patient with relapsed multiple myeloma after bone marrow transplantation: A case report. Clin Case Rep 2019;7:1426-9. [Crossref] [PubMed]
- Lane JW, Rehak NN, Hortin GL, et al. Pseudohyperphosphatemia associated with high-dose liposomal amphotericin B therapy. Clin Chim Acta 2008;387:145-9. [Crossref] [PubMed]
- Brownstein CA, Adler F, Nelson-Williams C, et al. A translocation causing increased alpha-klotho level results in hypophosphatemic rickets and hyperparathyroidism. Proc Natl Acad Sci U S A 2008;105:3455-60. [Crossref] [PubMed]
- Noda H, Guo J, Khatri A, et al. An Inverse Agonist Ligand of the PTH Receptor Partially Rescues Skeletal Defects in a Mouse Model of Jansen's Metaphyseal Chondrodysplasia. J Bone Miner Res 2020;35:540-9. [Crossref] [PubMed]
- Brown WW, Jüppner H, Langman CB, et al. Hypophosphatemia with elevations in serum fibroblast growth factor 23 in a child with Jansen's metaphyseal chondrodysplasia. J Clin Endocrinol Metab 2009;94:17-20. [Crossref] [PubMed]
- Höppner J, Kornak U, Sinningen K, et al. Autosomal recessive hypophosphatemic rickets type 2 (ARHR2) due to ENPP1-deficiency. Bone 2021;153:116111. [Crossref] [PubMed]
- Palma-Lara I, Pérez-Ramírez M, García Alonso-Themann P, et al. FAM20C Overview: Classic and Novel Targets, Pathogenic Variants and Raine Syndrome Phenotypes. Int J Mol Sci 2021;22:8039. [Crossref] [PubMed]
- Zheng WH, Yao Y, Zhou H, et al. Hyperphosphatemia and Outcomes in Critically Ill Patients: A Systematic Review and Meta-Analysis. Front Med (Lausanne) 2022;9:870637. [Crossref] [PubMed]
- Leung J, Crook M. Disorders of phosphate metabolism. J Clin Pathol 2019;72:741-7. [Crossref] [PubMed]
- Malberti F. Hyperphosphataemia: treatment options. Drugs 2013;73:673-88. [Crossref] [PubMed]
- Lippi G, Salvagno GL, Montagnana M, et al. Influence of hemolysis on routine clinical chemistry testing. Clin Chem Lab Med 2006;44:311-6. [Crossref] [PubMed]
- Albersen M, Bökenkamp A, Schotman H, et al. Hyperphosphatemia in an 11-year-old girl with acute myeloid leukemia: Answers. Pediatr Nephrol 2019;34:627-9. [Crossref] [PubMed]
- Amalnath SD, Dubashi B. Pseudohyperphosphatemia in Waldenstrom's Macroglobulinemia. Indian J Nephrol 2013;23:465-6. [Crossref] [PubMed]
- Bailey HL, Chan EM. Liposomal amphotericin B interferes with the phosphorus assay on the Synchron LX 20 analyzer. Clin Chem 2007;53:795-6. [Crossref] [PubMed]
- Bohm NM, Hoover KC, Wahlquist AE, et al. Case-control study and case series of pseudohyperphosphatemia during exposure to liposomal amphotericin B. Antimicrob Agents Chemother 2015;59:6816-23. [Crossref] [PubMed]
- Francis ER, Chen F, Kidacki M, et al. Pseudohyperphosphatemia in a patient with incidentally identified progression of smoldering myeloma. Clin Chim Acta 2018;487:306-8. [Crossref] [PubMed]
- Jensen GM, Bunch TH, Wolf S, et al. Erroneous determination of hyperphosphatemia (‘pseudohyperphosphatemia’) in sera of patients that have been treated with liposomal amphotericin B (AmBisome). Clin Chim Acta 2010;411:1900-5. [Crossref] [PubMed]
- Lee Y, Koo T, Yi JH, et al. Pseudohyperphosphatemia in a patient with multiple myeloma. Electrolyte Blood Press 2007;5:131-5. [Crossref] [PubMed]
- Mendoza D, Connors S, Lane C, et al. Liposomal amphotericin B as a cause of pseudohyperphosphatemia. Clin Infect Dis 2008;46:645-6. [Crossref] [PubMed]
- Miller MM, Johnson PN, Hagemann TM, et al. Pseudohyperphosphatemia in children treated with liposomal amphotericin B. Am J Health Syst Pharm 2014;71:1462-8. [Crossref] [PubMed]
- Sinclair D, Smith H, Woodhead P. Spurious hyperphosphataemia caused by an IgA paraprotein: a topic revisited. Ann Clin Biochem 2004;41:119-24. [Crossref] [PubMed]
- Stratta P, Canavese C, Quaglia M, et al. A patient with unexplained hyperphosphataemia. Nephrol Dial Transplant 2006;21:2664-6. [Crossref] [PubMed]
- El Bouchti I, Belkhou A, Younsi R, et al. Pseudohyperphosphatemia in multiple myeloma. Joint Bone Spine 2007;74:206-7. [Crossref] [PubMed]
- Aiyer R, Kazory A. Spurious hyperphosphatemia: a case for caution. Am J Kidney Dis 2012;60:1050-1. [Crossref] [PubMed]
- Loh TP, Saw S, Sethi SK. Hyperphosphatemia in a 56-year-old man with hypochondrial pain. Clin Chem 2010;56:892-5. [Crossref] [PubMed]
- Vaidya GN, Bhattad VB, Aggarwal A. Pseudohyperphosphatemia in Multiple Myeloma: a Commonly Misdiagnosed Phenomenon. Sci Postprint 2014;1:e00039. [Crossref]
- Talebi S, Gomez N, Iqbal Z, et al. Spurious Hyperphosphatemia: A Diagnostic and Therapeutic Challenge. Am J Med 2016;129:e15-6. [Crossref] [PubMed]
- Boud'hors C, Le Gallo M, Orvain C, et al. Hyperphosphatemia and Multiple Myeloma: Keep Calm and Control First. Am J Med 2020;133:e197-8. [Crossref] [PubMed]
- Chakraborty S, Kallner A. Measurement of serum-phosphate concentration in immunoglobulin G monoclonal gammopathy after PEG-precipitation. Clin Chim Acta 2015;440:211-3. [Crossref] [PubMed]
- Suchin EJ, Cizman B, Connolly BR, et al. Pseudohyperphosphatemia in a hyperphosphatemic hemodialysis patient. Am J Kidney Dis 2002;40:E18. [Crossref] [PubMed]
- Fernandes VPI, Lomazi Da Costa Pinto EA, De Fatima Santana Ferreira Boin I, et al. Phosphorus levels during infusion of parenteral nutrition with calorie-based phosphorus concentration: A case series. e-SPEN, the European e-Journal of Clinical Nutrition and Metabolism 2009;4:e252-6.
- Agarwal B, Walecka A, Shaw S, et al. Is parenteral phosphate replacement in the intensive care unit safe? Ther Apher Dial 2014;18:31-6. [Crossref] [PubMed]
- Marek I, Benz K, Kusnik S, et al. Phosphatintoxikation nach Klysmagabe – eine lebensgefährliche iatrogene Komplikation. Klin Pädiatr 2015;227:235-8. [Crossref] [PubMed]
- Casais MN, Rosa-Diez G, Pérez S, et al. Hyperphosphatemia after sodium phosphate laxatives in low risk patients: prospective study. World J Gastroenterol 2009;15:5960-5. [Crossref] [PubMed]
- Hamilton Smith R, Eddleston M, Bateman DN. Toxicity of phosphate enemas - an updated review. Clin Toxicol (Phila) 2022;60:672-80. [Crossref] [PubMed]
- Marcinowska-Suchowierska E, Kupisz-Urbańska M, Łukaszkiewicz J, et al. Vitamin D Toxicity-A Clinical Perspective. Front Endocrinol (Lausanne) 2018;9:550. [Crossref] [PubMed]
- Cernaro V, Longhitano E, Calabrese V, et al. Progress in pharmacotherapy for the treatment of hyperphosphatemia in renal failure. Expert Opin Pharmacother 2023;24:1737-46. [Crossref] [PubMed]
- Anand A, Aoyagi H. Understudied Hyperphosphatemia (Chronic Kidney Disease) Treatment Targets and New Biological Approaches. Medicina (Kaunas) 2023;59:959. [Crossref] [PubMed]
- Fujii N, Hamano T, Hsu JY, et al. A Comparative Study of Serum Phosphate and Related Parameters in Chronic Kidney Disease between the USA and Japan. Am J Nephrol 2022;53:226-39. [Crossref] [PubMed]
- St-Jules DE, Goldfarb DS, Pompeii ML, et al. Phosphate Additive Avoidance in Chronic Kidney Disease. Diabetes Spectr 2017;30:101-6. [Crossref] [PubMed]
- Kidney Disease: Improving Global Outcomes. Acute Kidney Injury (AKI). Kidney Int Suppl 2012;2:2.
- Flowers KC, Allen GT, Darragh-Hickey C, et al. Investigative algorithms for disorders affecting plasma potassium: a narrative review. J Lab Precis Med 2022;7:25. [Crossref]
- Linglart A, Levine MA, Jüppner H. Pseudohypoparathyroidism. Endocrinol Metab Clin North Am 2018;47:865-88. [Crossref] [PubMed]
- Mornet E, Hypophosphatasia . Metabolism 2018;82:142-55. [Crossref] [PubMed]
- Whyte MP, May JD, McAlister WH, et al. Vitamin B6 deficiency with normal plasma levels of pyridoxal 5′-phosphate in perinatal hypophosphatasia. Bone 2021;150:116007. [Crossref] [PubMed]
- Shajani-Yi Z, Ayala-Lopez N, Black M, et al. Urine phosphoethanolamine is a specific biomarker for hypophosphatasia in adults. Bone 2022;163:116504. [Crossref] [PubMed]
- Colantonio DA, Kyriakopoulou L, Chan MK, et al. Closing the Gaps in Pediatric Laboratory Reference Intervals: A CALIPER Database of 40 Biochemical Markers in a Healthy and Multiethnic Population of Children. Clin Chem 2012;58:854-68. [Crossref] [PubMed]
- Dorea JG. Calcium and phosphorus in human milk. Nutr Res 1999;19:709-39. [Crossref]
- Avidime O, Avidime S, Randawa AJ, et al. Physiological Changes in Serum Calcium, Phosphate, Vitamin D, Parathyroid Hormone and Calcitonin During Pregnancy and Lactation in Randomised Population of Zaria Women. Niger J Physiol Sci 2022;37:77-82. [Crossref] [PubMed]
- Møller UK, Streym S, Mosekilde L, et al. Changes in calcitropic hormones, bone markers and insulin-like growth factor I (IGF-I) during pregnancy and postpartum: a controlled cohort study. Osteoporos Int 2013;24:1307-20. [Crossref] [PubMed]
- Tung KTS, Wong RS, Cheung CKM, et al. Total 25(OH)D Concentration Moderates the Association between Caffeine Consumption and the Alkaline Phosphatase Level in Pregnant Women. Nutrients 2022;14:1616. [Crossref] [PubMed]
Cite this article as: Flowers KC, Shipman KE, Shipman AR, Gittoes NJL. Investigative algorithms for disorders causing hypophosphataemia and hyperphosphataemia: a narrative review. J Lab Precis Med 2024;9:33.